Sandia’s Sensor Microsystems effort develops sensors and sensor arrays for chemical, physical, and biological detection.
Custom Solutions
Microsensors
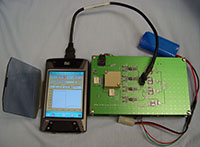
A particular class of devices known as shear horizontal surface (SH-SAW) sensors is well-suited for the detection of biological agents in liquid environments. These devices have the dual advantages of high sensitivity (down to picograms/cm2) and high specificity conferred by biological receptors such as antibodies, peptides, and nucleic acids. Sandia has demonstrated the detection of bacteria, viral particles, and proteins with these sensors. Handheld biodetection systems incorporating these microsensors are under development.
Click here for a technical overview.
Shear Horizontal Surface (SH-SAW) Video
Sandia has developed a miniature acoustic lysing system that rapidly releases nucleic acids and proteins from cellular samples, enabling more efficient DNA sample preparation. The technology provides a fast and reagentless method to produce a continuous source of lysate without harsh chemical agents. The present system uses plastic cartridges to couple the microchannel lysing regions to a standalone acoustic actuator array. The disposable microchannel cartridges are fabricated using layers of mylar and acrylic, which can be built-up to create complex 3D structures. When compared to commercial lysing instruments, the Sandia system provides higher efficiency, takes less time, consumes less power, and occupies a much smaller footprint. Sandia has further developed a DNA extraction method that uses magnetic-core beads with an electrostatic surface to bind and release DNA. This module integrates into the lysing system, providing polymerase chain reaction (PCR) quality DNA.
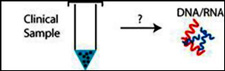
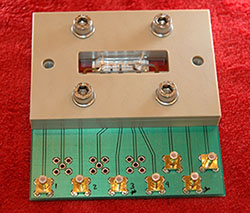
Sandia is developing a new battlefield adaptable agent detection platform capable of sensing multiple threat agents (chemical, biological and nuclear) simultaneously in diverse media, as well as a performance model of the detection platform. Magnetic microspheres impregnated with chromophoric dyes or Quantum Dots (QD) are used to develop sensitive tests for chem-bio-threat agents (CB-Agent). A variety of magnetic microspheres (µbeads) may be impregnated with QD or dyes for barcoding. The surfaces of the microspheres are functionalized with streptavidin protein. Biotinylated antibodies to various chem-bio-threat agents may then be stably anchored to the µbeads. The ratio of the optical signature (fluorescence, chemiluminescence or absorption) in the presence and absence of the chemical or biological agent is an indicator for a particular agent.
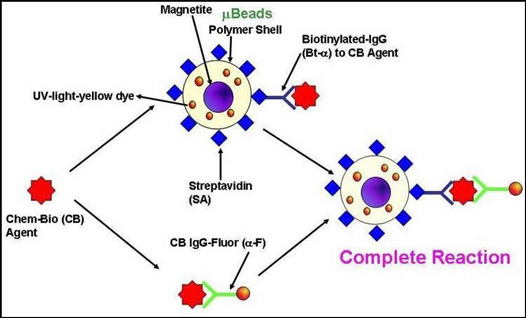
The reliable and definitive detection of multiple biowarfare agents on a single robust platform would be a significant asset for the defense of our nation and the safeguarding of warfighters. Multiple signature based biosensors can meet this need as they not only allow for multianalyte detection, but also substantially increase confidence in the sensor output as whole cell, genomic, and proteomic data can be interrogated for each target analyte. Sandia has developed a method that allows for controlled and selective immobilization of biorecognition elements onto electrodes, allowing simultaneous multianalyte detection of DNA and proteins.
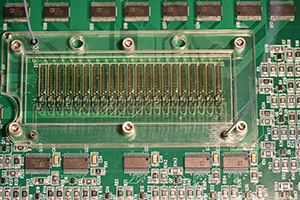
Overview – Microneedle Technology
- Microneedles are painless due to their size, avoiding deeper layers of the dermis where higher concentrations of nerves exist.
- Initially developed for drug delivery.
- Using microneedles for acquiring interstitial fluid to characterize physiological conditions has largely been ignored.
- Delivery and detection system feasible.
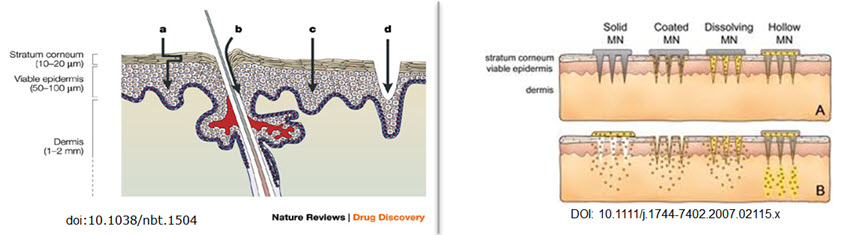
Microneedle Sensors for Defense of the Warfighter
- Microneedle-integrated electrode array combined with electrochemical detection.
- Device to be worn directly on skin for transdermal sensing.
- Continuously measure blood or interstitial fluid.
- Electronic readout.
- Multiplexed sensing platforms allows for site specific and systemic monitoring of critical injuries and essential biological markers.
- Device could be coupled with a microneedle drug delivery chip for an autonomous "sense-act-treat" system.
- Remote monitoring and treatment feasible.
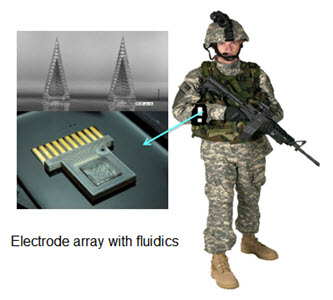
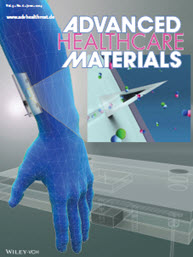
Advanced Healthcare Materials Article Cover
Click for cover abstract and full article.
Multiphoton Fabricated Microneedles
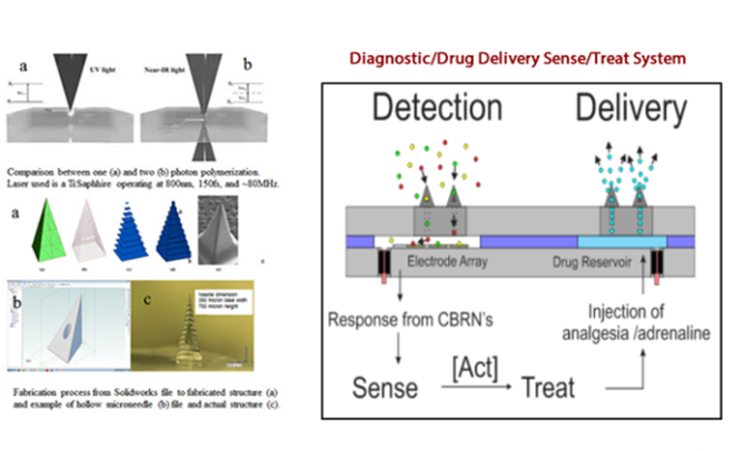
Sandia has developed genetically tailored cells and bio-compatible self-assembly approaches to enable whole cell sensors in patterned fluidic architectures for chemical and biological sensing. Combining genetics and molecular biology techniques, Sandia designed three reporter/promoter constructs that are integrated directly into yeast chromosomes that produce green, cyan, and red fluorescent protein in response to cholera toxin exposure.
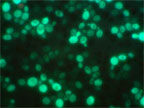
Geometries of nature are the subject of intense interest to chemists, biologists, physicists and mathematicians. Examples of natural geometries are found in living organisms (sea shells, for example) and among molecular components. Supra-molecular self-assembled aggregates yield interesting geometries through a collection of molecules held together by non-covalent bonds such as electrostatic forces, hydrogen bonding, or hydrophobic interactions, to provide homogeneous or heterogeneous assemblies. Certain cyanine dyes form molecular aggregates that are either the J- or H-type depending on their chemistry and sample milieu. H-aggregates have a blue-shifted absorption band, relative to the monomeric dye absorption wavelength. J-aggregates have a narrower, red-shifted absorption band, compared to the monomer; J-aggregates display sharp, intense fluorescence emission. Spectral properties of J- and H-aggregates make them attractive candidates for developing a variety of chem-bio-sensing applications.
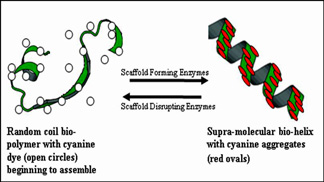
Sandia combined bio-assay technologies with its own state-of-art photonics technologies to develop a compact, planar, arrayable, optically based biosensor with unprecedented sensitivity. The sensor devices, fabricated in Sandia’s Microelectronics Development Laboratory, employ guided wave structures enhanced by the addition of ring-resonators. Overall sensitivity is further enhanced by using gold or quantum dot nanoparticles as the attached optical reporter tags to amplify the optical response, with a goal of being able to detect as little as one bound DNA strand.
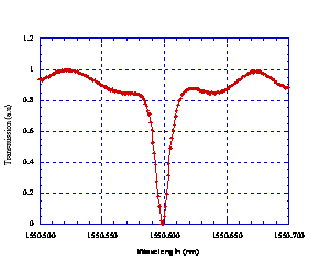
Diameter was 400 µm, cross-section was 1.2 µm x 0.2µm
A significant disadvantage of many biosensors is the requirement of labels or reagents for sensitive and specific biological detection. Sandia has shown that utilization of electrocatalytic nanoparticles allows for reagent-less and highly sensitive protein detection. Sandia is conducting work on electrocatalytic nanoparticles, focusing on the development of reagent-less and label-free methods for biological detection using the unique properties of nanoparticles.
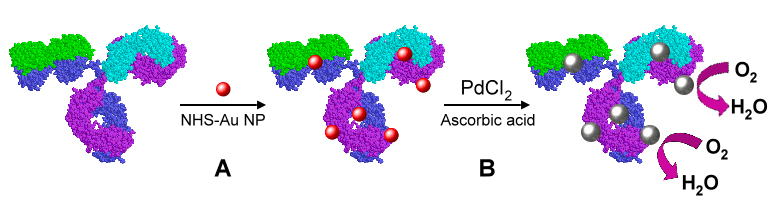
MicroFludics
Monitoring the immune response in single host cells challenged with pathogens is required in order to understand the stochasticity of immune response in a population of cells. Sandia has developed a microfluidic platform for optical interrogation of an array of single host cells. Additionally, Sandia has developed an electrofluidic platform for impedimetric interrogation of single host cells during pathogenic challenges.
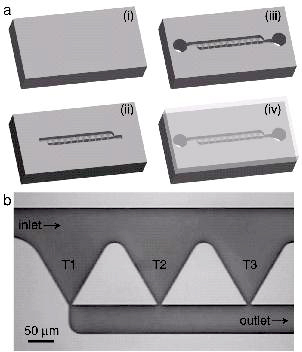
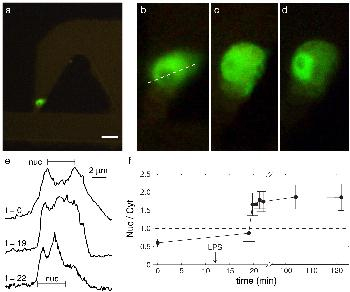
Figure 1: (left) Schematic of the (a) SCA chip fabrication and (b) optical micrograph of the chip. (right) (a-d) Macrophage held in a trap during an exposure to LPS. (e) Fluorescence intensity across the cell and (f) the ratio of nuclear to cytoplasm fluorescence as a function of time.
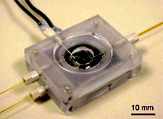
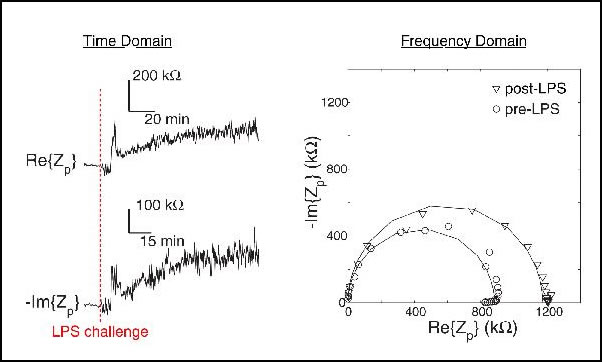
A large array of chemical sensors with responses interpreted using a pattern-recognition algorithm can discriminate a variety of chemicals. Chemiresistors, which are small, simple, sensitive, rugged microsensors with low power requirements capable of detecting chemical vapors in air, soil, or water, are low-cost devices that can be easily implemented in a sensor array. Sandia is examining a number of polymer/conductive particle combinations as chemiresistor materials in order to develop a flexible chemical sensor “micro-lab” for detecting volatile organic compounds. These chemiresistors can be incorporated in a badge-type device to protect first responders from chemical exposure, or serve as an environmental monitor for contamination (e.g., municipal water supplies).
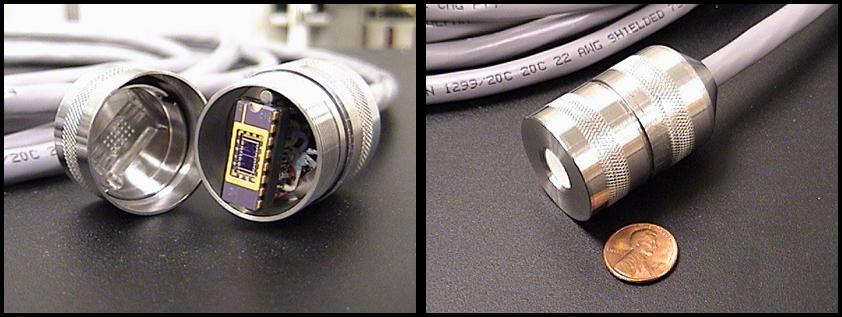
The combustible gas sensor consists of a conductive filament, coated with a catalytic layer that is heated by an electrical current. Micromachining technology allows the filament to be made very small and suspended above the substrate for thermal isolation, resulting in low-power operation. Combustible gases react on the catalytic surface, releasing heat that changes the filament resistance. This device is proposed for use in exhaust gas analysis and to indicate combustible gas hazards.
In collaboration with the University of Utah, MIT, and the University of New Mexico, Sandia National Laboratories’ Microsensors Sience & Technology department has modeled, designed, fabricated, and tested a combustible gas sensor that uses a resistively heated, noble metal-coated, micromachined polycrystalline silicon filament to detect the presence and concentration of combustible gases.
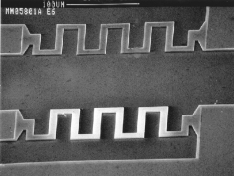
The detection and discrimination of nitroaromatic explosives are of considerable interest for national security issues and for environmental concerns related to the manufacturing, testing, and disposal of explosives. Sandia has demonstrated a novel multivariate data analysis approach that takes advantage of the partial selectivity of three separate glassy carbon surfaces towards the electrochemical discrimination of four different nitroaromatic compounds.
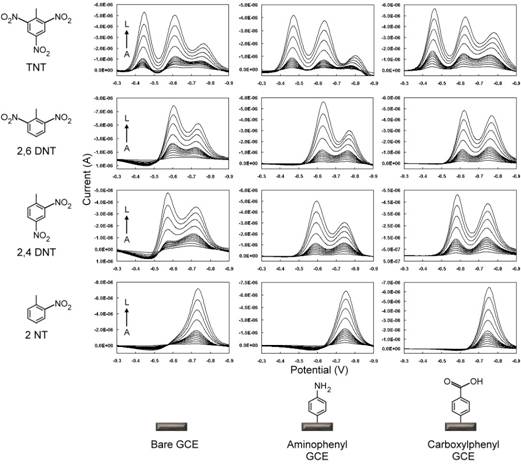
A new-generation hydrogen sensor that is smaller, faster, sturdier, and less expensive to manufacture has been developed at Sandia National Laboratories. Early versions of this practical, reliable device for detecting hydrogen already have completed the design, fabrication, and test stages and are now in field application.
Existing techniques for detecting hydrogen have numerous drawbacks: limited dynamic range; poor reproducibility and reversibility; and subjectivity to false alarms. Further, they are slow, unreliable, and difficult to use.
In comparison, the Sandia sensor provides:
- Hydrogen detection over a broader range of concentrations (.0001% to 100%).
- Smaller size to allow monitoring at various points (.4 cm3 size, 1 gram weight).
- Reliable performance over a greater temperature range (-100° C to 140° C).
- Dependable operation in diverse environments (vacuum, non-oxygen ambient, hostile vibration/radiation conditions).
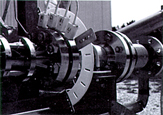
Sensors based on surface (SAW) devices are being developed to detect a wide range of chemicals. The SAW device is an extremely sensitive gravimetric detector that can be coated with a film to collect chemical species of interest. Based on these devices, sensor systems have been developed that can detect trace (ppm to ppb) levels of airborne contaminants. Applications include weapon state-of-health, environmental, and non-proliferation monitoring.
A new area of research and development at Sandia National Laboratories is exploring the utility of -based microsensors for high temperature gas monitoring. Robust coatings on SAW and bulk wave resonant devices allow sensitive measurement of gas species concentration at temperatures above 250°C. The small size, low cost, and simple implementation of these sensors make them excellent candidates for monitoring vehicle exhaust streams and industrial combustion processes.

The microhotplate, used as a basis for the microcombustor, is fabricated by through-wafer silicon etching. It consists of a silicon nitride membrane suspended from a frame of Si using either the Bosch etching or KOH etching to release the membrane, with no discernable operational differences between the completed devices made by either method.
Sandia National Laboratories is developing two gas sensors based on a microcombustor platform. While both sensors are in early stages of testing and development, they exhibit good detection limits and signal quality. In addition to testing the sensors themselves, headway has also been made in understanding the combustion characteristics of hydrocarbons when exposed to a variety of catalysts. The two sensor technologies are a micro flame ionization detector (microFID) and a calorimetric gas sensor. Both are utilized in the determination of fuel mixture, though the microFID has a demonstrated analyte set that expands beyond hydrocarbon detection.
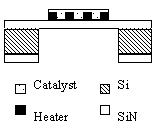
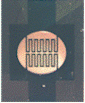
A novel integrated microsensor being developed at Sandia National Laboratories will enable increased sensitivity for trace detection and identification of a variety of chemical species. The sensor combines surface (SAW) technology with microelectronics to produce a monolithic, compact, low-power integrated circuit microsensor. The sensor is fabricated entirely using processing technology available in Sandia’s Center for Compound Semiconductor Science and Technology (CCSST).
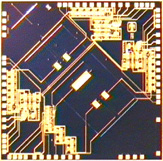
Ion mobility spectrometry (IMS) drift tubes fabricated from low-temperature co-fired ceramic (LTCC) materials and LIGA control structures hold promise for inexpensive mass-production of IMS-based sensor systems. Sandia National Laboratories has produced a hand-held explosives detection system based on these miniature drift tubes for first responder applications. Adequate sensor performance has been demonstrated while virtually eliminating the assembly and deployment complexity of traditional “stacked” IMS designs. In addition to explosive detection, the Sandia technology also has applications in the detection of biological material, chemical weapons, and drugs.
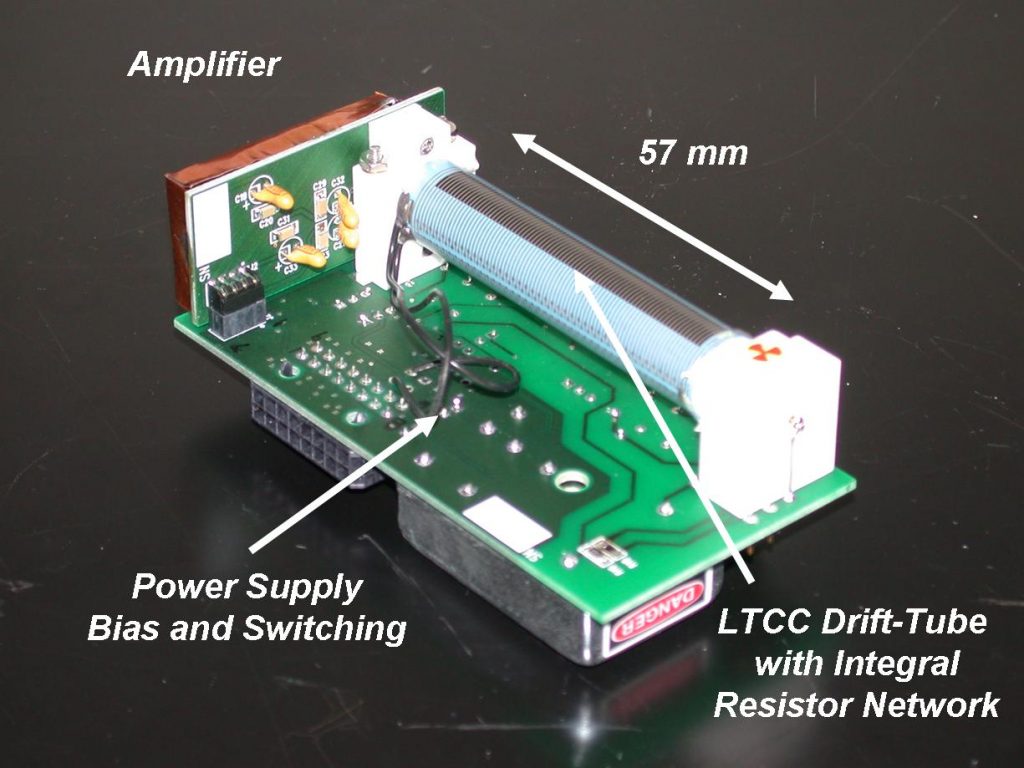
Sandia has developed small, potentially low-cost chemical sensors and sensor systems for nonproliferation and materials control applications, as well as for monitoring of chemical weapons threats. The most advanced of these systems is the MicroChemLab.
Sandia’s experience with the Department of Energy (DOE) sponsored nonproliferation programs and with the current accelerated needs of Homeland Defense programs clearly indicate a need for reliable, low cost, flexible, and potentially autonomous systems for field calibration and performance verification of such chemical sensors. These sensors can also be adapted for human health monitoring, detecting marker compounds or drug metabolites in human breath, urine, and blood.
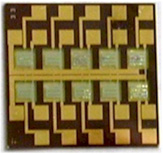
Breakthrough improvements in simplicity and reductions in the size of mass spectrometers are needed for high-consequence fieldable applications, including error-free detection of chemical/biological warfare agents, medical diagnoses, and explosives/contraband discovery. These improvements are most likely to be realized with the reconceptualization of the mass spectrometer, rather than by incremental steps towards miniaturization.
Microfabricated arrays of mass analyzers represent such a conceptual advance. A massively parallel array of micrometer-scaled mass analyzers on a chip has the potential to set the performance standard for hand-held sensors due to the inherit selectivity, sensitivity, and universal applicability of mass spectrometry as an analytical method.
Sandia has designed and built an ion trap mass analyzer consisting of 1 x 10 6 micron-sized cylindrical ion traps. Additionally, Sandia has microfabricated massive parallel ion trap arrays consisting of traps with r 0 = 1, 2, 5 and 10 µm. The instrument is the result of a conceptually radical change in the scaling of both size and number of ion traps and in the fabrication approach compared to previous embodiments.
Sandia is developing sensors that are responsive to electrochemical reactions to detect a wide range of chemicals in water. These sensors consist of highly ordered arrays of nanometer sized dots (electrodes) that detect trace (ppm to ppb) levels of waterborne contaminates. The design of the arrays takes advantage of diffusion phenomenons at the surface of tens of millions of tiny electrodes, which would be lost using larger electrodes. Applications include environmental, industrial, and domestic monitoring for surety and safety of water resources.
Click here for a technical overview.
Thin films composed of nanoparticles have emerged as useful chemical sensor platforms. These detection sensors have demonstrated the ability to sense various chemical agents with sensitivity in the sub part per million volume range. Nanoparticle-based sensors provide a simple signal transduction scheme based on changes in resistance. They also consume less energy and are easier to integrate into an embedded single chip platform.
Sandia is conducting a number of projects centering on nanoparticles for chemical sensing, including synthesis of nanoparticles and a sample preparation system.
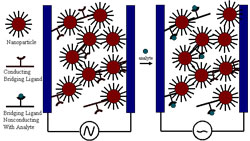
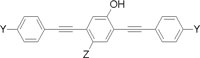
(Conjugated phenylene ethynylene)
Chemical species can be detected optically using optical correlation spectroscopy: broadband light passing through an unknown gas sample picks up spectral features of the sample and by correlating the light with known spectra, species in the sample can be identified. Sandia is working to develop programmable diffraction gratings that can synthesize molecular spectra for use in an optical correlation spectrometer. These consist of micromachined diffraction elements whose "weighting" can be varied. When broadband light is incident on the array, light reflected from the individual elements interferes to produce a desired spectrum.
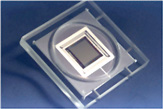
Sensors based on bulk quartz resonators are being developed to monitor fluid properties such as density and viscosity and to act as in situ chemical sensors for liquids. The device is electrically excited into a shear mode of vibration that probes the mechanical properties of a contacting fluid. The small, rugged device can be operated inside engines or pipelines to monitor properties of critical fluids such as lubricants.
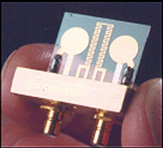
Sandia is developing sensors based on surface (SAW) devices to detect a wide range of chemicals. The SAW device is an extremely sensitive gravimetric detector that can be coated with a film to collect chemical species of interest. Based on these devices, sensor systems have been developed that can detect trace (ppm to ppb) levels of airborne contaminants. Applications include weapon state-of-health, environmental, and non-proliferation monitoring.
SAW-Based Chemical Sensors Video
Smart Sand uses a distributed chemical particle with a fluorescent signature and LIDAR detection. Sandia developed this technology to address the growing international problem of unrecovered land mines causing harm to people long after hostilities have ceased. The protocol sequence used to detect land mines is as follows:
- Crop "dust" suspected areas with sensor particles
- Particles react with target chemical to produce a fluorescent signature.
- Detection LIDAR or fluorescence imaging method.

Sandia has developed "multi-region", fiber-optic, gas-phase surface plasmon resonance sensors (SPRS) for the detection of corrosive and reactive off-gassing from materials confined in sealed systems. These sensors are single-ended, requiring only one penetration of the system, and can be placed in various regions of interest in the sealed system since they are fiber-based. In addition, multiple sensor regions allow speciation and quantization of several target species on a single fiber. To predict corrosion of sensitive electronics and electronic connections in sealed systems, Sandia has demonstrated H2O, H2, and sulfur compound sensitivity on a single 600 µm core optical fiber.
Sandia has fabricated and tested highly sensitive sensors for monitoring the effects of corrosion. The onset and progression of corrosion is monitored through changes in sensor resistance, capacitance, impedance, or resonant frequency.
Devices with progressively stronger resistance to corrosion can be used in parallel to determine the corrosive environmental history of susceptible nearby components.
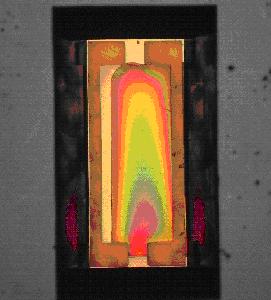
Figure 1: Corrosion Sensor
Sandia is developing a novel measurement technique that uses surface (SAW) devices and passive radio frequency (RF) to measure the spatial temperature profile of a body such as a satellite or aero shell. Variation of the SAW characteristic delay and center frequency allow the manufacture of inexpensive, robust, self-identifying sensor element arrays that can be read using a single antenna and data acquisition system. This wireless temperature sensor allows for large arrays of self-identifying sensors to be pre-positioned for measurement of the structure, and does not require batteries that add unacceptable mass to the structure or wires to retrieve the data.
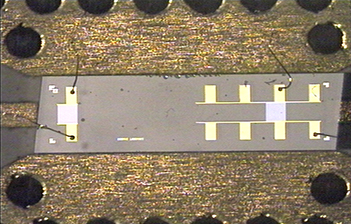
For more information please visit the MicroElectroMechanical Systems (MEMS) and Quantum Systems pages.
Optical Micro Sensors
Optical Detection of Biowarfare Agents – Overview
Guided wave devices are a unique technology used to interrogate fluid properties and operate as high density immunoassays for detection of biowarfare agents. Sandia has designed and fabricated a Mach-Zehnder interferometer with a microring attached to one arm to measure the induced phase change by the ring resonator. Sandia has also designed a fluidic microchannel that permits selected delivery of two distinct fluids to the microresonator rings.
Sandia is a leader in event-driven readout integrated circuit (ROIC) concepts, driven mostly by Sandia’s Global Burst Detection (GBD) mission area. Unlike traditional FPA applications that read out every pixel in every frame, this particular application requires high-speed sampling and assessment of sparse events. This requirement has driven innovation at Sandia in the area of efficient state-of-the-art, event-driven ROICs which can rapidly parse massive datasets and identify events of interest.
Sensor Microsystems
Passive sensors interrogated by 1 mW “radar guns” give 8-bit accuracy at 10 meters with omni-directional antennas. These sensors are often used to instrument a complex system after deployment when it is difficult to tap into power and data buses but there is an apparent need for more monitoring.
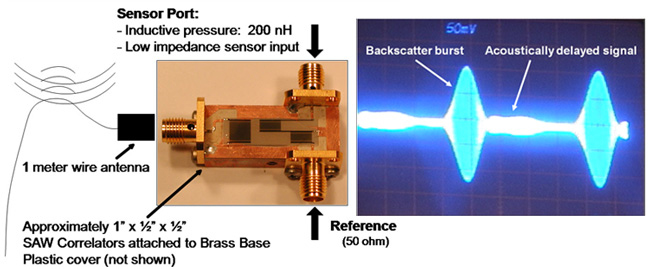
Sandia has developed a dielectrophoretic (DEP) gate for manipulating suspended particles in liquids using electric fields. Differences in polarizability between the particle and the fluid leads to body-forces on the particles that enable preconcentration, routing, and transport of particles in fluids. Lateral transport can also be accomplished by shifting electric-fields in an array of interdigitated microelectrodes. The Sandia technology has applications in repairing damaged neural tissue.
Sensor Animations
R&D
The Research and Development website is currently under development.
Fact Sheets, Publications, References, Animations, Licensing IP Opportunities
Physical Microsensors
Sensors Publications
Polsky, R., Harper, J. C., Brozik, S. M. (2010). “Electrochemical Signaling with Modified Surfaces and Nanoparticles”, in The Supramolecular Chemistry of Organic-Inorganic Hybrid Materials. pp. 273-296. Hoboken, New Jersey: John Wiley & Sons, Inc.
Dolan, P. L., Harper, J. C., Brozik, S. M. (2006). “Microbial Detection Systems”, in Encyclopedia of Medical Devices and Instrumentation. 2nd ed. Vol. 4, pp. 373-383. Hoboken, New Jersey: John Wiley & Sons, Inc.
K.E. Achyuthan, P.D. Adams, B.A. Simmons & A.K. Singh, “Hitherto Unrecognized Fluorescence Properties of Coniferyl Alcohol”, MOLECULES, 15: 1645-1667, 2010.
Y. Tang, K.E. Achyuthan & D.G. Whitten, “Label-free and Real-Time DNA Sequence Detection Based on Supramolecular Self-assembly” Langmuir, 2010 (In Press).
K.E. Achyuthan, D.G. Whitten & D.W. Branch, “Supramolecular Self-Assembling Cyanine as an Alternative to Ethidium Bromide Displacement in DNA-Drug Model Interactions during High Throughput Screening”, Analytical Sci. 26: 55-61, 2010.
K.B. Pfeifer, S. M. Thornberg, “Multi-Component Fiber Optic Surface Plasmon Sensor for Gas Phase Contaminants”, The 28th Compatibility, Aging and Stockpile Stewardship Conference, Sept. 29-Oct. 2, 2009, Albuquerque, NM.
K.B. Pfeifer, S. M. Thornberg, “Surface Plasmon Sensing of Gas Phase Contaminants Using Optical Fiber”, SAND2009-6096 September 2009.
K.E. Achyuthan, P.D. Adams, B.A. Simmons & A.K. Singh, “Spectroscopic Analyses of the Biofuels-critical Phytochemical, Coniferyl Alcohol and its Enzyme-catalyzed Oxidation products”, MOLECULES 14: 4758-4778, 2009.
Z. Zhou, Y. Tang, D. G. Whitten & K. E. Achyuthan, “A new High-throughput Screening Protease Assay based upon Supramolecular Self-Assembly”, Appl. Mater. Interfaces, 1: 162-170, 2009.
K.E. Achyuthan, J.L. McClain, Z. Zhou, D.G. Whitten & D.W. Branch. “Spectroscopic Analyses of the Noncovalent Self-Assembly of Cyanines Upon Various Nucleic Acid Scaffolds”, Analyt. Sci., 25: 469-474, 2009.
K.E. Achyuthan, J.L. McClain & D. Raj. “Orthogonal, Spectroscopic High-throughput Screening of Laccase-Catalyzed p-Cresol Oxidation”, Comb. Chem. HTS., 12: 678-689, 2009.
K.E. Achyuthan, D.C. Arango, E.L. Carles, C.E. Cutler, L.A. Meyer & S.M. Brozik. “Luminescent Investigations of Terbium (III) Biosorption as a Surrogate for Heavy Metals and Radionuclides”, Mol. Cell. Biochem., 327: 87-92, 2009.
Polsky R, Stork CL, Wheeler DR, Steen WA, Harper JC, Washburn CM, Brozik SM, “Multivariate Analysis for the Electrochemical Discrimination and Quantitation of Nitroaromatic Explosives”, Electroanalysis, 21, No. 3-5, 550 – 556, 2009.
Davis RW, Arango DC, Jones HDT, Van Benthem MH, Haaland DM, Brozik SM, Sinclair MB, “Antimicrobial peptide interactions with silica bead supported bilayers and E. coli: buforin II, magainin II, and arenicin”, J. Pept. Sci., 15: 511–522, 2009.
K. Ogawa, K.E. Achyuthan, S. Chemburu, E. Ji, Y. Liu, G. P. Lopez, K.S. Schanze & D.G. Whitten. “Polyelectrolyte Based Fluorescent Sensors”, In: Organic Semiconductors in Sensor Applications, Eds. D. Bernards, R. Owens & G. Malliaras, Springer, pp 39-60, 2008.
Komandoor Achyuthan, "Photophysical, Chemical and Optical Investigations of Self-Assembled Nanomaterials," SAND2007-6635, Pub Date: October 2007.
Manginell, Ronald Paul; Moorman, Matthew Wallace; Bauer, Joseph M.; Adkins, Douglas Ray; Lewis, Patrick Raymond; Hadizadeh, Rameen; Copic, Davor; Porter, Daniel Allen; Norton, Blaine Eric; Hietala, Vincent Mark; Bryan, Jon R.; Wheeler, David Roger; Pfeifer, Kent Bryant; Rumpf, Arthur Norman; Siegal, Michael P.; “SMART micro-preconcentrator for integrated preconcentration and detection of chemical agents and explosives”, SAND2007-6467, October, 2007.
K.E. Achyuthan & D.G. Whitten. “Design Considerations for High-throughput Screening and In Vitro Diagnostic Assays”, Comb. Chem. HTS. 10: 399-412, 2007.
Davis RW, Flores A, Barrick TA, Cox JM, Brozik SM, Lopez GP, and Brozik JA, “Nanoporous Microbead Supported Bilayers: Stability, Physical Characterization, and Incorporation of Functional Transmembrane Proteins”, Langmuir, 23, 3864-3872, 2007.
Atanassov P, Apblett C, Banta S, Brozik S, Barton SC, Cooney M, Liaw BY, Mukerjee S, Minteer SD, “Enzymatic biofuel cells”, Electrochemical Society Interface, 16(2), 28-31, 2007.
David Roger Wheeler, Shawn M. Dirk, William Kent Schubert, G. Ronal Anderson and Paul Martin Baca, "Polymer electronic devices and materials", SAND2006-6723, November 2006.
Dulce C. Arango, Susan M. Brozik, Patricia L. Dolan, Jason C. Harper and Monica Manginell, "Viral Vectors for Gene Modification of Plants as Chem/Bio Sensors", SAND2006-6955, October 2006.
C. Jeffrey Brinker, David B. Burckel, Shawn M. Dirk, Hongyou Fan, Stephen W. Howell, Brandi K. Price, Robert J. Simonson, Brian S. Swartzentruber and David R. Wheeler,"Engineering Conjugated Molecule-Linked Metal Nanocrystal/Silica Arrays for Integrated Chemical Platforms: Final LDRD Report", SAND2006-6884, October 2006.
Conrad D. James, Mark Steven Derzon, Kenneth Roy Pohl, Komandoor Achyuthan and Jaime McClain, "Quantification of false positive reduction in nucleic acid purification on hemorrhagic fever DNA", SAND2006-6922, November 2006.
Harold G. Craighead, Joshua B. Edel, Grant D. Meyer, Jose C. Moran-Mirabal, Daniel J. Throckmorton, "Lipid Microarray Biosensor for Biotoxin Detection", SAND2006-2981, May 2006.
Pfeifer, K. B., Flemming, J. H., Sinclair, M. B., “Detection of carbon monoxide (CO) as a furnace byproduct using a rotating mask spectrometer”, SAND2007-0667, February 2006.
K.E. Achyuthan, L. Lu, G.P. Lopez & D.G. Whitten. “Supramolecular Photochemical Self-Assemblies For Fluorescence “Turn On” and “Turn Off” Assays For Chem-Bio-Helices”, Photochem. Photobiol. Sci. 5: 931-937, 2006.
D.G. Whitten, K.E. Achyuthan, G.P. Lopez & O-K. Kim. “Cooperative Self-Assembly Of Cyanines On Carboxymethylamylose And Other Anionic Scaffolds As Tools For Fluorescence-Based Biochemical Sensing”, Pure & Appl. Chem. 78: 2313-2323, 2006.
Yelton, William Graham, Siegal, Michael P.; Pfeifer, Kent Bryant “Functionalized nanoelectrode arrays for in-situ identification and quantification of regulated chemicals in water.”, EWRI-World Water & Environmental Resources Congress 2005 held on May 15-19, 2005 in Anchorage, AK.
C. D. Mowry, L. A. Theisen, P. R. Lewis, D. R. Wheeler, R. W. Cernosek, K. B. Pfeifer, “Low Power Microfabricated Components for Rapid and Portable Detection of Biological Agents”, First Annual National Homeland Security R&D Conference, Feb. 27-28, 2005, Boston, MA.
Yelton, W. G., Pfeifer, K. B., Brevnov, D. A. , Gurule, N. J., Kelly, M. J., Ashby, C. I., “Nano Electrode Arrays for In-situ Identification and Quantification of Chemicals in Water”, Sand 2004-6229, December 2004.
J. J. Allen, M. B. Sinclair, K. B Pfeifer, “Advanced polychromator systems for remote chemical sensing : (LDRD project 52575)”, SAND2004-6700, December 2004.
Sinclair, Michael B>, Pfeifer, Kent Bryant, “Chemical sensing with a MEMS-based correlation radiometer”, SAND2004-1793A, American Ceramic Society Fall Meeting held November 7-12, 2004 in Cocoa Beach, FL.
Pfeifer, Kent Bryant, Sinclair, Michael B., ‘Stand-off chemical sensing using MEMS-based correlation radiometry”, SAND2004-4426A, Sixth Joint Conference on Standoff Detection for Chemical and Biological Defense held October, 25-29, 2004 in Williamsburg, VA
Mowry, Curtis Dale; Theisen, Lisa Anne; Goodin, Andrew M; Pfeifer , Kent B; “Detection of triacetone triperoxide (TATP) using commercial and portable ion mobility spectrometers.”, SAND2004-0748A, American Society of Mass Spectrometry Conference on Mass Spectrometry and Allied Topics held May 24-27, 2004 in Nashville, TN.
Sinclair, Michael B., ; Pfeifer , Kent B; , Butler, Michael Alan Polychromix, Inc., Woburn, MA, Senturia, Stephen D. Polychromix, Inc., Woburn, MA, Deutsch, Erik R. Polychromix, Inc., Woburn, MA, Youngner, Dan W. Honeywell Technology Center, Plymouth, MN, Cabuz, Eugen Honeywell Technology Center, Plymouth, MN, Hocker, G. Benjamin Honeywell Technology Center, Plymouth, MN, “A MEMS-based correlation radiometer.”, SAND2003-4470C, Photonics West 04 held January 24-29, 2004 in San Jose, CA.
Davis RW, Patrick EL, Meyer LA, Ortiz TP, Marshall JA, Keller DJ, Brozik SM, and Brozik JA, “The Thermodynamic Properties of Single Ion Channel Formation: Gramicidin”, J. Physical Chem., 108, no. 30, 15364-15369, 2004.
Timlin JA, Sinclair MB, Haaland DM, Martinez MJ, Manginell M, Brozik SM, Guzowski JF, Werner-Washburne M, “Hyperspectral imaging of biological targets: The difference a high resolution spectral dimension and multivariate analysis can make”, IEEE International Symposium on Biomedical Imaging: Macro to Nano; 2, 1529-1532, 2004.
Daryl J. Dagel, James J. Allen, Jeffrey L. Dohner, Kent B. Pfeifer, Michael B. Sinclair, Olga Blum Spahn, Christine M Wehlburg, and Joseph C. Wehlburg, “MEMS Adaptive Optics Devices: LDRD No. 02-1385 Summary Report”, SAND 2002-2954, January 2003.
W. Graham Yelton, Kent B. Pfeifer, Alan W. Staton, “Porous Al2O3 Nanogeometry sensor films: Growth and Analysis”, Journal of the ElectroChemical Society, V. 149(#1) pp. H1-H5, Jan 2002.
K. B. Pfeifer, R. W. Cernosek, A. N. Rumpf, R. L. Waldschmidt, “An Examination Of System Architectures for Distributing Sensor Data Via Ethernet Networks”, SAND2000-3228, (Jan. 2001)
Robert C. Hughes, W. Graham Yelton, Kent B. Pfeifer, Sanjay V. Patel, “Characteristics and Mechanisms in Ion-Conducting Polymer Films as Chemical Sensors”, Journal of the Electrochemical Society, V 148(#4), pp. H37-H44, Apr. 2001.
Kent B. Pfeifer, Sarah K. Leming, Arthur N. Rumpf, “Embedded Self-Powered Microsensors for Monitoring the Surety of Critical Buildings and Infrastructures”, SAND2001-3619.
Kelly, Michael James; Goehner, Raymond P.; Blewer, Robert S.; Stallard, Brian R.; Garcia, Manuel J.; Pfeifer, Kent B.; Guilinger, Terry Rae; Peterson, David W.; Tuck, Melanie R.; Sweet, James N.; Edwards, Victoria A.; Zavadil, Kevin R.; Cordes, Gary T.; “Sensors for the detection of moisture in inert and corrosive gases”, Semiconductor Industry Developments and Results from the Twelve years of the Ultraclean Society by The Society for the Study of Semiconductor Substrate Technology SAND2001-0271P
W.G. Yelton, K. Pfeifer, and A. Staton, “Porous Al2O3 Nano Geometry Sensor Films: Part I Anodization of Vacuum Evaporated Aluminum Formed Under Zone 2 Growth”, Electrochemical Society Meeting, Toronto, ON, May 2000.
K.B. Pfeifer and W.G. Yelton, “Porous Al2O3 Nano Geometry Sensor Films: Part II Heat of Adsorption Studies”, Electrochemical Society Meeting, Toronto, ON, May 2000.
K. B. Pfeifer, R. L. Waldschmidt, “Capacitive-Based Stress Sensors for Tire Application”, SAND99-2961 (Nov. 1999).
Robert C. Hughes, W. Graham Yelton, Sanjay V. Patel, Kent B. Pfeifer, “Conducting Polymer Films as Chemical Sensors”, Chemical Sensors IV, 17-22 Oct. 1999, Honolulu, HI.
K. B. Pfeifer, T. J. Rudnick, “Design, Fabrication, and Implementation of Capacitive Type Sensors for Computer Aided Fabric Evaluation (CAFÉ), SAND99-1686 (July 1999).
“Ion-Conducting Polymer Films as Chemical Sensors”, APS Centennial Meeting, Atlanta, GA, March 22, 1999.
Kent B. Pfeifer, Robert L. Jarecki, and Timothy J. Dalton, “Fiber-Optic Polymer Residue Monitor”, SPIE International Symposium on Industrial and Environmental Monitors and Biosensors, Boston, MA Nov. 1-6, 1998.
K. B. Pfeifer, R. L. Jarecki, and T. J. Dalton, “Fiber-optic Polymer Residue Monitor”, SPIE Conference on Chemical Microsensors and Applications, Boston, MA, Vol. 3539, Nov. 1998.
K. B. Pfeifer, R. C. Hughes, and M. W. Jenkins, “Viologen Polymer Coated Impedance Sensors for Midrange Moisture Sensing”, ECS Meeting, Montreal, Quebec, Canada, 05/04/97. SAND97-0015A.
K. B. Pfeifer, “Recent Microsensor Research for Semiconductor Applications at Sandia National Laboratories”, Texas Instruments visit, 11/14/96, Dallas, TX. SAND97 0900A
K. B. Pfeifer, “NMP Measurement Using Devices: NCAICM Final Report”, SAND96-1866 (1996).
K. B. Pfeifer, “Calibration Procedures For High Sensitivity Chemical Sensors”, SAND95-2055 (September 1995).
K. B. Pfeifer, an Overview of Film Based Microsensors and Applications”, Adhesion Society Meeting, Myrtle Beach, SC, February 18-21, 1996, SAND95 148A.
G. C. Frye, R. W. Cernosek, S. J. Martin and K. B. Pfeifer, “The Simple and Rapid Technique For Monitoring Phase and Amplitude Changes In Sensors”, Electrochemical Society Meeting, Miami Beach, FL, October 9-14, 1994
K. B. Pfeifer, M. J. Kelly, T. R. Guilinger, D. W. Peterson, J. N. Sweet, and M. R. Tuck, “Development of Solid State Moisture Sensors for Semiconductor Fabrication Applications”, Microcontamination, San Jose, CA Oct. 4-6, 1994.
K. B. Pfeifer, “Surface Trace Moisture Sensor for HCI Process Gas”, Contamination Control Strategies Symposium, Austin, Texas, 2/7 11/94
K. B. Pfeifer, J. L. Sprung and J. R. Galloway, “CCL4 Monitoring In Steam Reforming Environments”, Electrochemical Society Meeting, New Orleans, LA, 10/10 15/93.
K. B. Pfeifer, S. J. Martin and A. J. Ricco, “Surface Sensing of VOC’s in Harsh Chemical Environments”, SAND93-0070 (June 1993)
R. C Hughes, A. J. Ricco, M. A. Butler and K. B. Pfeifer, “The Detection of Organophosphonates by Polymer Films On a Surface Device and a Micromirror Fiber Optic Sensor”, Applied Biochemistry and Biotechnology, Vol 38, 1993. (8/26/92).
R. C Hughes, A. J. Ricco, M. A. Butler and K. B. Pfeifer, “Detection of Organophosphonates by Polymer Films On a Surface Device and a Micromirror Fiber Optic Sensor”, Microfabrication of Biosensors Seminar, Anchorage, Alaska, 7/21 24/92.
G.C. Frye, S. J. Martin,R. W. Cernosek, K. B. Pfeifer and J. S. Anderson, “ Sensor Systems”, Proceedings of 1991 IEEE Ultrasonics Symposium, Lake Buena Vista, FL, 12/8-11/91.
M. A. Butler, K. B. Pfeifer and A. J. Martin, “Two Dimensional Barker Patterns for Optical Alignment”, Applied Optics, Vol. 30, No. 32, 10 Nov. 1991.
K. B. Pfeifer, “In Situ Monitoring of Thin Film Deposition Using Optical Fibers”, SAND91-1407 (September 1991)
K. B. Pfeifer and M. A. Butler, “A Fiber Optic Weight Monitor For Intrusion Sensing Applications”, SAND89-2274 (November 1989).
K. B. Pfeifer and M. W. Jenkins, “A Fiber Optic Test System For Quantum Efficiency Measurements”, SAND89-0593 (May 1989).
K. B. Pfeifer, “A Fiber Optic Synchronous Detector Using Sample-And-Hold Circuits”, SAND88-0389 (February 1988).