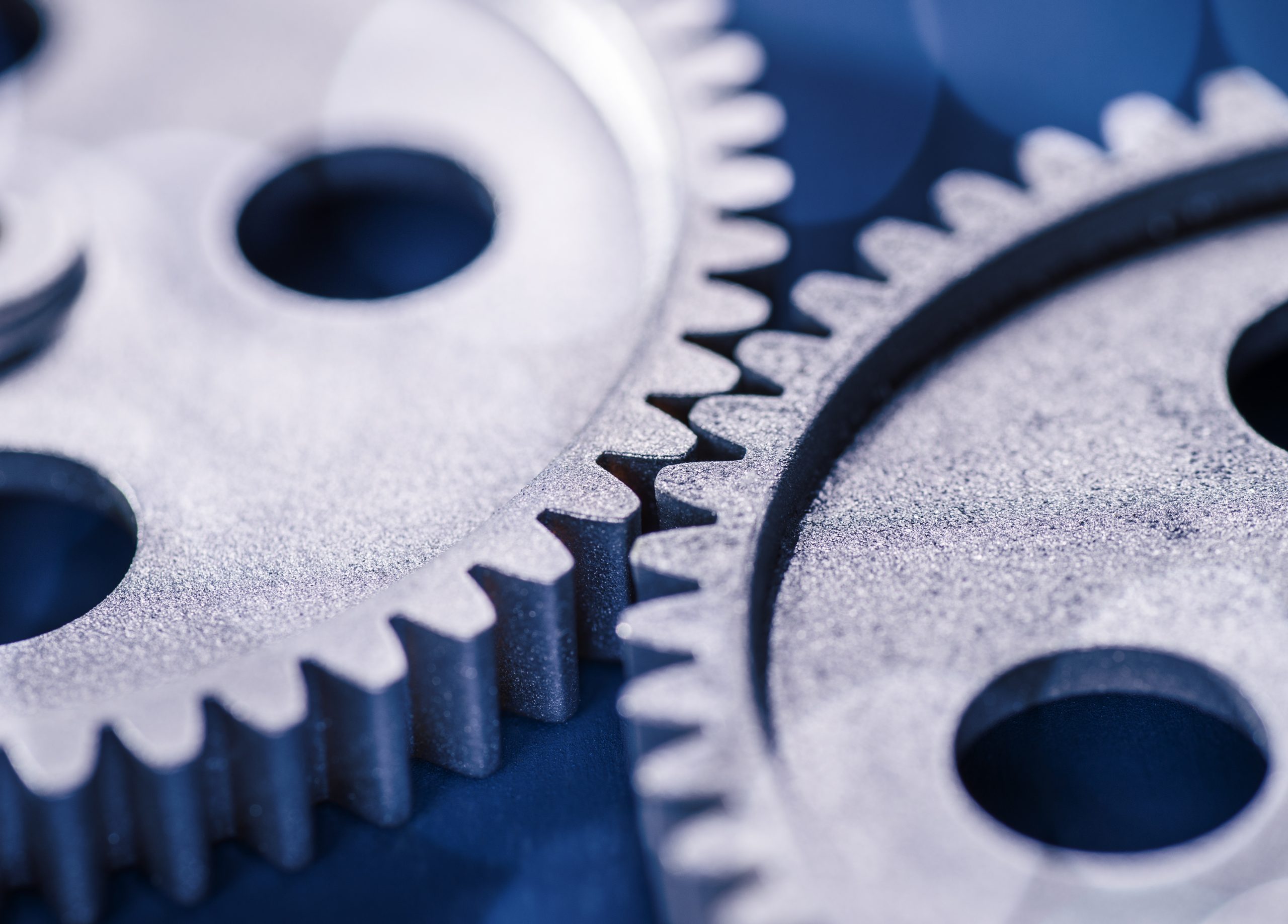
MicroElectroMechanical Systems (MEMS)
The MEMS Technology Department at Sandia National Laboratories conducts research and development for advanced microelectromechanical systems that push the technology envelope for national security applications
Custom Solutions
Inertial & Pressure Sensors
Inertial sensors have emerged as the most significant MEMS product in consumer electronics. Sandia develops MEMS inertial sensors with an emphasis on nuclear and space environments where radiation hardness is required.
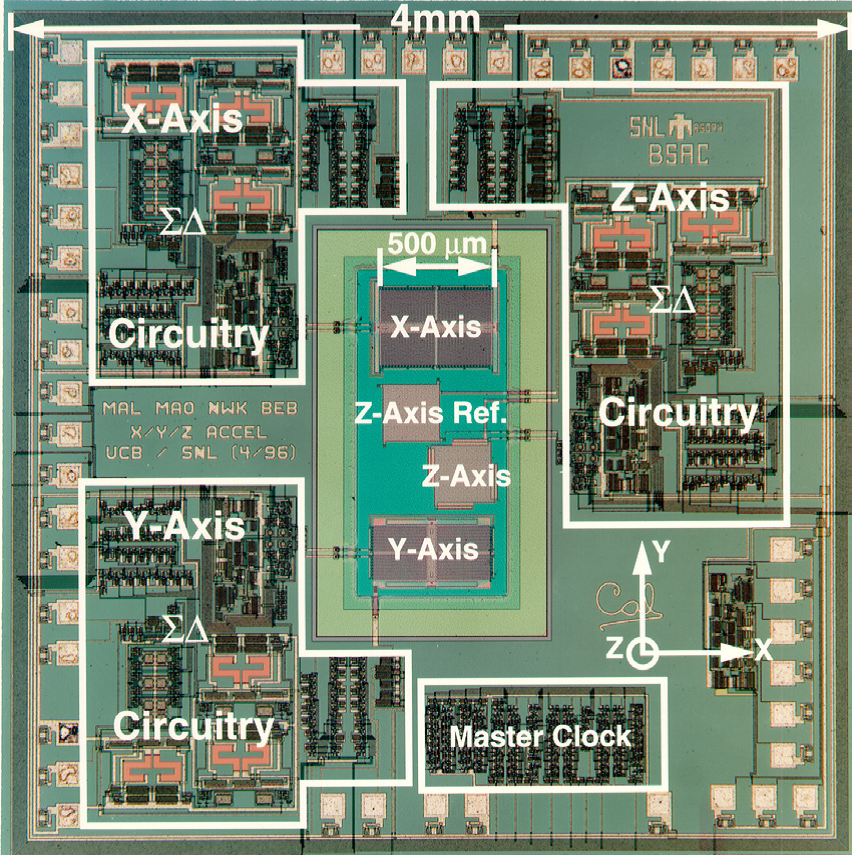
Microelectromechanical system (MEMS) sensors, including accelerometers, gyroscopes, pressure sensors, and microphones, have become a multi-billion dollar market in consumer electronics, automobile, and industrial applications. Sandia helped lay the foundation for these sensors in the 1990s, when the labs fabricated early exploratory designs for accelerometers and gyroscopes from the University of California, Berkeley. Sandia also demonstrated a MEMS-first fabrication process for integrating MEMS with supporting complementary metal–oxide–semiconductor (CMOS) electronics on the same chip. Today, Sandia fills niches in national security, where commercial devices are not available because the technology is too immature or the market is too small.
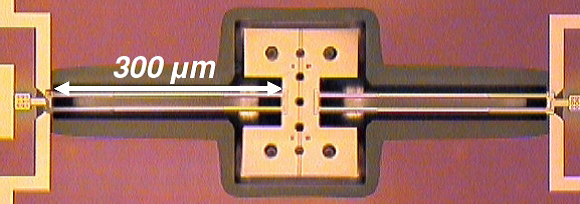
Sandia focuses on inertial sensors, such as accelerometers and gyroscopes, that perform in radiation environments. Because the signals are small, a MEMS sensor must be close to its supporting electronics. Sandia develops accelerometers and gyroscopes together with radiation-hard CMOS-7 electronics. One technical path being pursued is the integration of aluminum nitride piezoelectric resonant beam sensors on a CMOS-7 chip above the circuits. The aluminum nitride fabrication process is also used to make radio frequency filters and oscillators at multiple frequencies on the same chip.
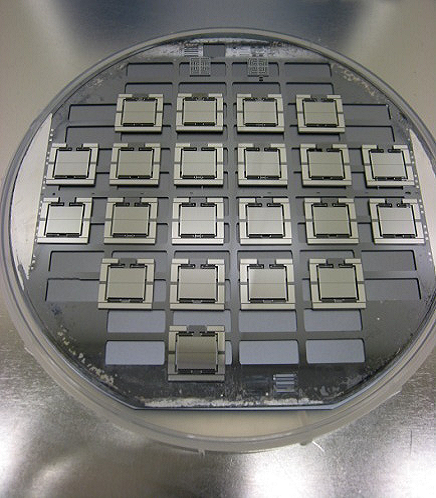
Sandia draws upon its many technologies to make high performance inertial sensors using complex fabrication schemes, which would be uneconomical for commercial applications. For example, an accelerometer for low amplitude seismic motions requires a large proof mass and the measurement of extremely small displacements, as well as ruggedness and stability. Deep reactive ion etching completely through the silicon wafer creates the proof mass, or the known quantity of mass used as a reference for measuring the unknown quantity. Optical interferometry, as is used for calibration standards, measures the displacement. Surface micromachined locks hold the proof mass when not conducting measurements, making the sensor rugged. Single crystal silicon springs that do not creep lead to long-term stability. The MEMS sensing mass is integrated with a stable laser, optics, gratings, photodiodes, and electronics to make the complete sensor.
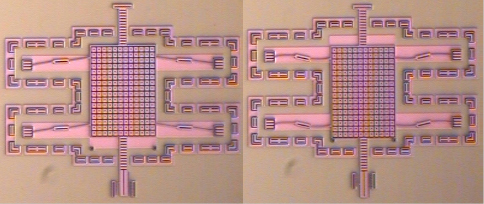
Some applications require only the indication that a specified inertial environment has occurred, for example a rocket achieving a certain velocity. Another example is the sensing of a pyrotechnic event, for which Sandia designed and fabricated a switch with a compliant mechanism that is stable in two positions (bi-stable). The switch is moved from electrically open to closed only when the pyrotechnic shockwave accelerates the proof mass. It can be integrated with a surface acoustic wave (SAW) device and radio antenna so that its state can be interrogated wirelessly. The sensor is totally passive, drawing no power.
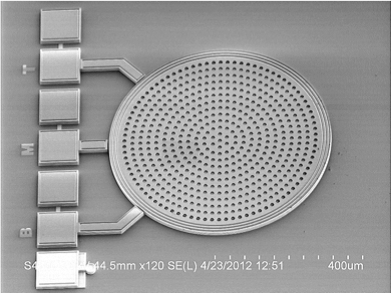
In an inertial sensor, a proof mass is moved by acceleration. In a pressure sensor or a microphone, a diaphragm is moved by pressure. Sandia develops pressure sensors for various uncommon environments, such as munitions penetrating into the ground. For example, Sandia fabricated an aeroacoustic microphone designed by the University of Florida, Gainesville. The microphone’s unique design incorporates capacitive sensing of diaphragm position using dual backplates, top and bottom. Its performance is comparable to expensive, industry-standard condenser microphones.
Electronics & RF MEMS
Commercial electronic products are beginning incorporate MEMS oscillators and clocks. Sandia is developing the next generation of MEMS electronic devices, namely radio frequency filters. Sandia has developed a fabrication technology using aluminum nitride for piezoelectric transducers that can be integrated with CMOS electronics. With the Sandia technology, radio frequency (RF) filter frequency characteristics are determined by photolithographically defined geometries rather than film thicknesses. This enables whole filter banks to be fabricated on a single chip.
Sandia designs, characterizes and delivers miniaturized, reliable RF MEMS components and systems such as filters, switches, and oscillators that provide differentiating capabilities to Sandia system groups impacting national security and nuclear weapons.
Aluminum Nitride RF MEMS Resonators
Sandia has developed an aluminum nitride (AlN) based microresonator RF MEMS process for realizing high performance, ultra-miniature RF filters and oscillators over a very wide frequency range. Microresonators are miniature acoustic resonators fabricated using integrated circuit (IC) microfabrication techniques. Aluminum nitride (AlN) microresonators are a promising technology for next generation cognitive radios because they enable many low insertion loss filters and oscillators spanning a wide frequency range (32 kHz – 10 GHz) to be fabricated in a small size on a single IC chip. In addition, microresonator filters and oscillators can be monolithically integrated with transistor electronics such as switches and amplifiers circuits for the realization of single chip radios. These properties allow for the realization of miniature programmable center frequency filters and oscillators spanning the HF to X-band range commonly used for communications.
Sandia wafer-level packaging technology ensures a very small final form factor and can be implemented in surface mount or chip and wire assemblies. Sandia AlN microresonators have demonstrated high levels of both stability and survivability over environmental conditions such as extreme temperatures, shock and vibration.
Because of its high temperature stability, high quality factor and extremely small footprint, Sandia microresonator technology is ideal for narrow band filtering applications where temperature stability, insertion loss and size are critical.
Finally, because it is capable of integration with CMOS electronics such as switches, Sandia’s AlN microresonator technology is an ideal choice for applications requiring reconfigurable center frequency, reconfigurable bandwidth, or very wide spectral coverage.
To learn more about Sandia Aluminum Nitride Microresonators, please click on the links below or read one of Sandia’s many publications.
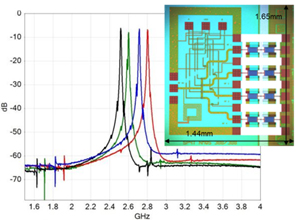
Integrated with CMOS.
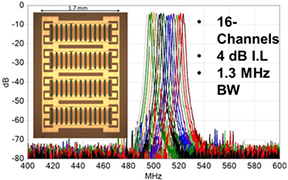
Micro Fluidic & MEMS Actuators
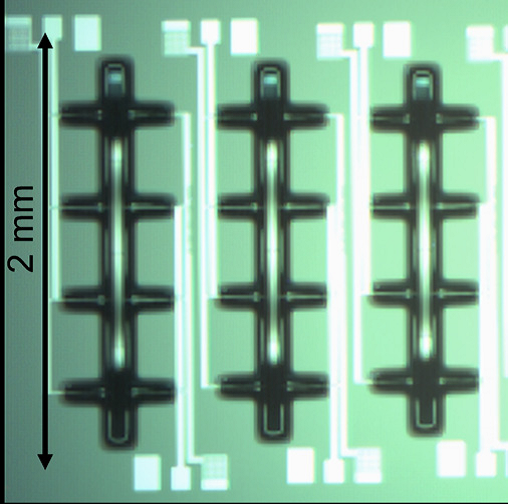
The common inkjet printer uses MEMS microfluidic devices to precisely dispense picoliter drops of ink. Sandia has fabricated a number of microfluidic devices, including inkjet dispensers and micro-nozzles for experimental jet propulsion. For another application, Sandia’s high-pressure valves enable the two-stage Micro Gas Analyzer (MGA) by precisely controlling high-pressure gas sample flow with an extremely low leak rate and without the use of polymer coatings in the seals.
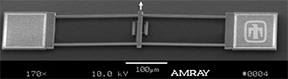
Thermal Actuators
The V-shaped thermal actuator shown in the figure below is commonly referred to as a “chevron” or “bent-beam” thermal actuator. It is used in applications requiring high force and reliability. These actuators are based on the constrained thermal expansion of the angled beams (a result of Joule heating when a current is passed through the legs of the actuator), resulting in motion of the center shuttle in the direction shown by the arrow in the figure.
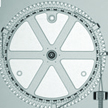
Thermal Ratcheting Actuator
The Thermal Ratcheting Actuator (ThRA) is a V-shaped thermal actuator attached to a ratcheting hub that provides a drive capable of high-force 360+ degree rotational motion.
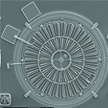
Torsional Ratcheting Actuator (TRA) The Torsional Ratcheting Actuator is used in applications requiring high-torque rotational motion. Ratcheting allows unidirectional 360+ degree motion.
Parallel Plate Actuation Electrostatic parallel-plate actuation is used extensively in RF MEMS, oscillators, resonators, mirrors, switches, and other applications requiring only small displacements. These actuators can be designed using simple equations.
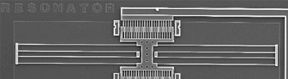
Comb Drives
The electrostatic “comb-drive” is a common MEMS actuator used in gyroscopes, microengines, resonators, and many other MEMS applications. The force output is generally less than 50 uN, but the linear and highly predictive behavior (very good predictions can be made using simple equations) make it a popular MEMS actuator. Comb drives have been used in microengines by combining the actuator with a rotary transmission.
Optical MEMS
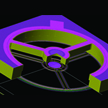
Sandia designs, develops, builds and delivers highly sensitive, reliable micro and nano-scale optical solutions across electromechanical and biological domains for physical sensing and optical signal processing in national security applications.
Sandia uses microfabrication techniques to create novel approaches and methodologies for micro- and nano-scale optical sensing and control. Sandia utilizes these approaches alongside proven opto-electro-mechanical techniques to push performance limits in displacement and acceleration sensing, optical wavefront control and beam shaping. These capabilities are applied with system level input to build high performance, reliable, integrated systems such as inertial and acoustic sensors, high speed optical switches and modulators, adaptive optics systems, micro-spectrometers, integrated optical circuits and on-chip fluorescence-based sensors for microfluidic systems.
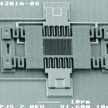
MEMS Optical Microphone
Optical MEMS microphone arrays offer extreme fidelity to size ratio. Owing to the precision of micromachining technology, the elements in these arrays have precisely matched dynamic frequency response. This combination makes the technology ideal for integration with advanced signal processing algorithms for directional sensing and source localization using sub cm 2 aperture systems.
Nanophotonic Optical Transducers for displacement Sensing
Sub-wavelength grating structures are coupled in the near field. As a result, very small changes in lateral displacement are detectable as changes in optical reflectance. This effect was first predicted and observed at Sandia Labs. Practical designs are being implemented to realize a new class of highly sensitive accelerometer devices.
Photovoltaic Cells and Radiation Detection
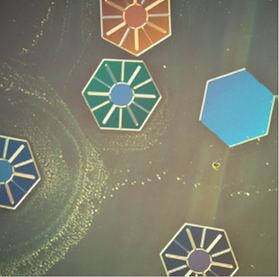
As Sandia has explored the application of MEMS technologies to new problems, it has discovered disruptive new approaches to solving old problems. For example, in traditional radiation sensors, radiation interacts with matter to create free charged particles that must travel long distances to be collected. Sandia inverted this paradigm by making the detecting volume small and integrating it with electrodes; then making large arrays of these pixels. Similarly, the interactions of photons in a photovoltaic (PV) cell occur in the tens of microns near the surface; the rest of the silicon wafer thickness is unnecessary for functionality. Sandia is developing PV cell technology where individual micro-sized cells are released from the substrate wafer, assembled into arbitrary arrays, and individually connected. This leads to extremely efficient use of expensive single crystal silicon and many system-level advantages.
R&D
The Research and Development website is currently under development.
Fact Sheets, Publications, References, Animations, Licensing IP Opportunities
MEMS Video & Image Gallery
MEMS Publications
[1] Baker, M.S., Plass, R.A., Headley, T.J. and Walraven, J.A., “Final Report: Compliant Thermo-Mechanical MEMS Actuators LDRD #52553,” Sandia Report SAND2004-6635, printed December 2004.
[2] E. J. Garcia and J. J. Sniegowski, “Surface Micromachined Microengine,” Sensors and Actuators A, Vol. 48 (1995) 203-214.
[3] Baker, Michael S. “Design and Reliability of a MEMS Thermal Rotary Actuator,” TEXMEMS IX, Texas Tech University, Lubbock TX, September 17, 2007.
[4] Tanner, D.M., Owen, A.C., Jr., and Rodriguez, F., 2003, “Resonant Frequency Method for Monitoring MEMS Fabrication,” Proceedings of SPIE – The International Society for Optical Engineering, Vol. 4980, pp. 220-228.
More Literature on MEMS Actuators …
J.H. Comtois, V.M. Bright, M.W. Phipps, “Thermal microactuators for surface-micromachining processes”, Proc. SPIE 2642 (1995) 10–21.
L. Que, J.-S. Park, Y.B. Gianchandani, “Bent-beam electro-thermal actuators for high force applications”, Proceedings of the IEEE Micro Electro Mechanical Systems, 1999, pp. 31–36.
R. Cragun, L.L. Howell, “Linear thermomechanical microactuators”, Proceedings of the IMECE, ASME International Mechanical Engineering Congress & Exposition, November, 1999, pp. 181–188.
B. Kim, R. H. Olsson III and K. E. Wojciechowski, “AlN Microresonator-Based Filters with Multiple Bandwidths at Low Intermediate Frequencies,” IEEE Journal of Microelectromechanical Systems, In-Press, 2013.
B. Kim, J. Nguyen, K. E. Wojciechowski and R. H. Olsson III, “Oven-Based Thermally Tunable Aluminum Nitride Microresonators,” IEEE Journal of Microelectromechanical Systems, In-Press, 2013.
G. Piazza, V. Felmetsger, P. Muralt, R. H. Olsson III and R. Ruby, “Piezoelectric Aluminum Nitride Films for Microelectromechanical Systems,” MRS Bulletin, Vol. 37, pp.1051 – 1061, Nov. 2012. (Invited Review)
C. D. Nordquist, R. H. Olsson III, S. M. Scott, D. W. Branch, T. Pluym, and V. Yarberry, “On/Off Micro-Electromechanical Switching of AlN Piezoelectric Resonators,” IEEE International Microwave Symposium, June 2013, In-Press.
R. H. Olsson III, E. Crespin, C. D. Nordquist, P. Clews and K. E. Wojciechowski, “Aluminum Nitride Micromechanical Resonators Monolithically Integrated with CMOS Electronics,” PiezoMEMS Workshop, April 2013, In-Press. (Invited)
R. H. Olsson III, J. Nguyen and T. Pluym, “A Programmable Bandwidth Aluminum Nitride Microresonator Filter,” Govt. Microcircuit App. and Critical Tech. Conf., March 2013, In-Press.
R. H. Olsson III, B. Kim, J. Nguyen, P. Clews, T. Pluym and K. E. Wojciechowski, “Tuning the Bandwidth and Center Frequency of Micromechanical Acoustic Resonators,” National Radio Science Meeting, Jan. 2013. (Invited)
E. R. Crespin, R. H. Olsson III, K. E. Wojciechowski, D. W. Branch, P. Clews, R. Hurley and J. Gutierrez, “Fully Integrated Switchable Filter Banks,” IEEE International Microwave Symposium, pp. 1-3, June 2012.
M. D. Henry, K. D. Greth, J. Nguyen, C. D. Nordquist, R. Shul, M. Wiwi, T. A. Plut and R. H. Olsson III, “Hermetic Wafer-Level Packaging for RF MEMs: Effects on Resonator Performance,” IEEE Electronic Components and Technology Conf., pp. 362-369, May 2012.
M. Ziaei-Moayyed, P. Clews, J. Nguyen and R. H. Olsson III, “C-Band Aluminum Nitride Resonator and Filter Arrays,” Govt. Microcircuit App. and Critical Tech. Conf., pp. 237-240, March 2012.
M. S. Baker, R. H. Olsson III and J. R. Schwank, “Radiation Testing of Aluminum Nitride Microresonators,” Govt. Microcircuit App. and Critical Tech. Conf., pp. 389-392, March 2012.
B. Kim, R. H. Olsson III, K. Smart and K. E. Wojciechowski, “MEMS Resonators with Extremely Low Vibration and Shock Sensitivity,” IEEE Sensors Conf., pp. 606-609, Oct. 2011. (Invited)
C. D. Nordquist and R. H. Olsson III, “Power Handling and Intermodulation Distortion of Contour-Mode AlN MEMS Resonators and Filters,” IEEE International Microwave Symposium, 978-1-61284-757-3/11 June 2011.
B. Kim, R. H. Olsson and K. E. Wojciechowski, “Capacitive Frequency Tuning of AlN Micromechanical Resonators,” 2011 16th International Solid-State Sensors, Actuators and Microsystems Conference (TRANSDUCERS), pp.502-505, June 2011.
R. Chanchani, C. D. Nordquist, R. H. Olsson III, T. Peterson, R. Shul, C. Ahlers, T. A. Plut and G. Patrizi, “A New Wafer-Level Packaging Technology for MEMS with Hermetic Micro-Environment,” IEEE Electronic Components and Technology Conference,” pp. 1604-1609, 2011.
R. H. Olsson III, D. W. Branch and K. E. Wojciechowski, “Origins and Mitigation of Spurious Modes in Aluminum Nitride Microresonators,” IEEE Ultrasonics Symposium, pp. 1272-1276, Oct. 2010.
B. Kim, R. H. Olsson III and K. E. Wojciechowski, “Ovenized and Thermally Tunable Aluminum Nitride Microresonators,” IEEE Ultrasonics Symposium, pp. 974-978, Oct. 2010.
R. H. Olsson III, K. E. Wojciechowski, M. Ziaei-Moayyed, B. Kim, J. E. Stevens, M. R. Tuck and C. D. Nordquist, “Aluminum Nitride Microresonator Filters and Oscillators for Defense and Consumer Radio Frequency Electronics,” Commercialization of Micro-Nano Systems Conf., Albuquerque, NM, Aug. 2010. (Invited)
K. E. Wojciechowski and R .H. Olsson III, “Parallel Lattice Filters Utilizing Aluminum Nitride Contour Mode Resonators,” Solid-State Sensor, Actuator, and Microsystems Workshop, pp. 65-69, June 2010.
R. H. Olsson III, K. E. Wojciechowski, J. E. Stevens, M. R. Tuck and C. D. Nordquist, “Aluminum Nitride Micro-Devices for RF Communications and Sensing,” U.S. Navy Workshop on Acoustic Transduction Materials and Devices, State College, PA, May 2010. (Invited)
K. E Wojciechowski, R. H. Olsson III, C. D. Nordquist, M. R. Tuck, and J. E. Stevens, “Next Frontier for MEMS-IC Integration: Aluminum Nitride (AlN) Frequency References, Filters and Sensors… Drivers and Challenges,” 8th Annual MEPTEC MEMS Symposium, San Jose, CA, May 2010. (Invited)
R. H. Olsson III, K. E. Wojciechowski, M. R. Tuck, J. E. Stevens and C. D. Nordquist, “Multi-Frequency Aluminum Nitride Micro-Filters for Advanced RF Communications,” Govt. Microcircuit App. and Critical Tech. Conf., pp. 257-260, March 2010.
D. M. Tanner, R. H. Olsson III, T. B. Parson, S. M. Crouch, J. A. Walraven and J. A. Ohlhausen, “Stability Experiments on MEMS Aluminum Nitride RF Resonators,” Proc. Of SPIE, vol. 7592, pp. 1-8, Feb. 2010.
R. H. Olsson III and K. E. Wojciechowski, “Microresonant Impedance Transformers,” IEEE Ultrasonics Symposium, pp. 2153-2157, Sept. 2009.
K. E. Wojciechowski, R. H. Olsson III and M. R. Tuck, “Super High Frequency Width Extensional Aluminum Nitride MEMS Resonators, IEEE Ultrasonics Symposium, pp. 1179-1182, Sept. 2009.
K. E. Wojciechowski, R. H. Olsson, T. A. Hill, M. R. Tuck and E. Roherty-Osmun, “Single-Chip Precision Oscillators Based on Multi-Frequency, High-Q Aluminum Nitride MEMS Resonators,” IEEE International Solid-State Sensors, Actuators and Microsystems Conference, pp. 2126-2130, June, 2009.
R. H. Olsson III and M. R. Tuck, “Fundamental and Overtone Aluminum Nitride Dual Mode Resonator Filters,” Solid-State Sensor, Actuator, and Microsystems Workshop, pp. 356-359, June 2008.
K. E. Wojciechowski, R. H. Olsson III and M. R. Tuck, “Post-CMOS Compatible Aluminum Nitride Ring Wave Guide (RWG) Resonators,” Solid-State Sensor, Actuator, and Microsystems Workshop, pp. 372-375, June 2008.
R. H. Olsson III, C. M. Washburn, J. E. Stevens, M. R. Tuck and C. D. Nordquist, “VHF and UHF Mechanically Coupled Aluminum Nitride MEMS Filters,” IEEE Frequency Control Symposium, pp. 634-639, June 2008.
R. H. Olsson III, J. G. Fleming, K. E. Wojciechowski, M. S. Baker and M. R. Tuck, “Post-CMOS Compatible Aluminum Nitride MEMS Filters and Resonant Sensors,” IEEE Frequency Control Symposium, pp. 412-419, June 2007. (Invited)
K. E. Wojciechowski, R. H. Olsson III, M. S. Baker, J. W. Wittwer, K. Smart, J. G. Fleming and K. R. Pohl, “Low Vibration Sensitivity MEMS Resonators,” IEEE Frequency Control Symposium, pp. 1220-1224, June 2007.
K. J. Smart, R. H. Olsson III, D. Ho, D. R. Heine, and J. G. Fleming, “Frequency Agile Radios Using MEMS Resonators,” Govt. Microcircuit App. and Critical Tech. Conf., pp. 409-412, March 2007.