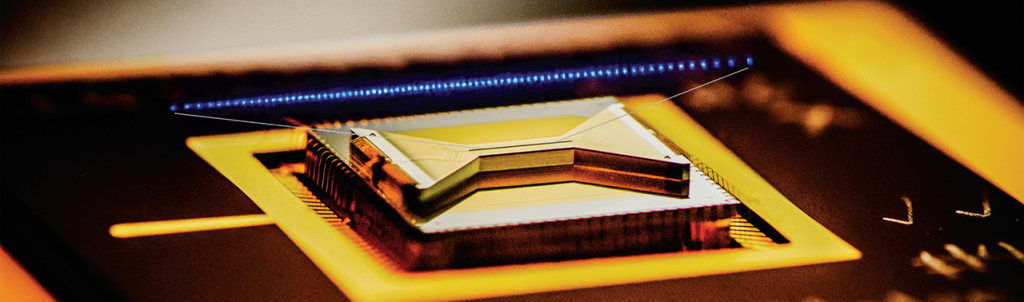
Try this thought experiment: imagine a small measurable quantity of something—perhaps a grain of sand or a point of light—then cut it in half, again and again. When you reach the smallest quantity, such as a photon of light, you will have created an indivisible quantum particle. Scientists and engineers can control these subatomic particles, or qubits, to design, build, and test revolutionary computers to answer extremely complex scientific questions. Sandia National Laboratories’ Quantum Scientific Computing Open User Testbed (QSCOUT) project takes on both challenges.
The five-year, $25.1 million QSCOUT project funded by the U.S. Department of Energy (DOE) Office of Science Advanced Scientific Computing Research (ASCR) program is advancing research on trapped ion quantum computer technology, one of the major competing technologies for producing full-scale quantum computers. Scientists in an ideal quantum computing world could access processing power exponentially greater than what is available now, even with supercomputers, to solve special classes of exascale problems. Already they are studying multidimensional problems involving simultaneous computation, communication, and sensing (global cybersecurity, for example) using complex mathematical processes known as algorithms.
Processing such volumes of data in a matter of seconds requires a new type of information science. QSCOUT aims to meet this need by providing unrestricted access to quantum hardware internals— the quantum gates and underlying pulse sequences— enabling users to adapt and modify them. Sandia will eventually support and advise users in the functionality of the QSCOUT testbeds, allowing them to realize the potential of high-fidelity quantum computing in a way not yet available to most researchers.
A major challenge confronts quantum technology: the qubits it relies on can be unstable and easily disturbed by the slightest changes in their environment. When that happens, they may experience decoherence, the process whereby they decay and eventually disappear. According to Sandia researcher and QSCOUT project lead, Peter Maunz, trapped ion technology has enormous potential to avoid the decoherence (also called noise) and gate error problems that make it difficult to build and to operate a working quantum computer with integrated quantum circuits.
Maunz explains: “Because trapped ions are identical and suspended by electric fields in a vacuum, they feature identical, nearly perfect qubits well isolated from the noise of the environment and able, therefore, to store and process information faithfully.” Further, he says, “While current small-scale quantum computers without quantum error correction are still noisy devices, quantum gates with the lowest noise have been realized with trapped-ion technology.”
With its focus on trapped ion quantum computer technology, QSCOUT builds on Sandia’s physics and engineering expertise in fabricating microelectromechanical systems and complementary metal oxide semiconductor devices at the Labs’ Microsystems and Engineering Sciences Applications (MESA) facility.
QSCOUT will also assess the potential of near-term quantum hardware by giving researchers access to a trapped-ion quantum computer to address scientific computing applications of interest to other DOE and DOE ASCR projects and programs. With orders of magnitude more power as they gain qubits, quantum computers will help researchers create and optimize complex algorithms that take advantage of quantum computing.
Sandia researcher linked to work
- Peter Maunz
Sponsored by

About QSCOUT
“The goal of QSCOUT is to build, maintain, and provide access to a quantum processor based on trapped ions to the larger scientific community” – Susan Clark, QSCOUT Principal Investigator, Sandia National Laboratories
Quantum Technologies for Discovery Science
Associated Publications
-
Muller, R. (2022). Sandia QIS Program Overview [Slides]. https://doi.org/10.2172/1885883 Publication ID: 80136
-
Yuan, H.-B., Bao, W.-T., Lee, C.H., Zinser, B.F., Campione, S., Lee, J.-F., & Lee, J.-F. (2022). A Method of Moments Wide Band Adaptive Rational Interpolation Method for High-Quality Factor Resonant Cavities. IEEE Transactions on Antennas and Propagation, 70(5), pp. 3595-3604. https://doi.org/10.1109/tap.2022.3142281 Publication ID: 76952
-
Sarma, R., Xu, J., de Ceglia, D., Carletti, L., Campione, S., Klem, J.F., Sinclair, M., Belkin, M., Brener, I., & Brener, I. (2022). An All-Dielectric Polaritonic Metasurface with a Giant Nonlinear Optical Response. Nano Letters, 22(3), pp. 896-903. https://doi.org/10.1021/acs.nanolett.1c03325 Publication ID: 80306
-
Warne, L., Campione, S., San Martin, L., Pack, A., Langston, W., Zinser, B., & Zinser, B. (2022). Penetration Bounds For Azimuthal Slot On Infinite Cylinder With Finite Length Backing Cylindrical Cavity. https://doi.org/10.2172/1854569 Publication ID: 79887
-
Campione, S., Warne, L., & Warne, L. (2021). Penetration through Slots in Overmoded Cavities. IEEE Transactions on Electromagnetic Compatibility, 63(6), pp. 1904-1909. https://doi.org/10.1109/temc.2021.3067005 Publication ID: 77837
-
Campione, S., Stephens, J., Martin, N., Eckert, A., Warne, L., Huerta, J., Pfeiffer, R.A., Jones, A., & Jones, A. (2021). Developing Uncertainty Quantification Strategies in Electromagnetic Problems Involving Highly Resonant Cavities. Journal of Verification, Validation and Uncertainty Quantification, 6(4). https://doi.org/10.1115/1.4051906 Publication ID: 79164
-
Muller, R. (2021). Quantum Computing: NISQ and Beyond [Conference Presenation]. https://doi.org/10.2172/1893286 Publication ID: 76320
-
Muller, R. (2021). Quantum Systems Accelerator [Presentation]. https://www.osti.gov/biblio/1888715 Publication ID: 75859
-
Sarma, R., Xu, J., de Ceglia, D., Carletti, L., Campione, S., Klem, J.F., Sinclair, M.B., Belkin, B., Mesh, M., Brener, I., & Brener, I. (2021). Control of Second-Harmonic Generation in All-Dielectric Polaritonic Metasurfaces via Microscopic Control of χ(2) [Conference Presenation]. https://doi.org/10.2172/1868865 Publication ID: 78474
-
Koepke, J., Ivie, J., Campbell, Q., Brickson, M., Schultz, P., Muller, R., Baczewski, A., Mounce, A., Bussmann, E., Misra, S., & Misra, S. (2021). Stochastic atomistic disorder in atomic-precision doping [Conference Presenation]. https://doi.org/10.2172/1855714 Publication ID: 77610
-
Freno, B., Johnson, W., Zinser, B.F., Wilton, D.R., Vipiana, F., Campione, S., & Campione, S. (2021). Characterization and integration of the singular test integrals in the method‐of‐moments implementation of the electric‐field integral equation. Engineering Analysis with Boundary Elements, 124, pp. 185-193. https://doi.org/10.1016/j.enganabound.2020.12.015 Publication ID: 75057
-
Brickson, M., Campbell, Q., Ivie, J., Koepke, J., Schultz, P., Muller, R., Bussmann, E., Baczewski, A., Mounce, A., Misra, S., & Misra, S. (2021). Signatures of missing donors in transport through atomically precise P donor chains in Si [Conference Presenation]. https://doi.org/10.2172/1854311 Publication ID: 77453
-
Campbell, Q., Ivie, J., Koepke, J., Brickson, M., Schultz, P., Muller, R., Bussmann, E., Baczewski, A., Mounce, A., Misra, S., & Misra, S. (2021). A chemical model for atomic-precision single-donor incorporation of phosphorus atoms in Si(100)-2×1 [Conference Presenation]. https://doi.org/10.2172/1854314 Publication ID: 77455
-
Freno, B., Johnson, W., Zinser, B.F., Wilton, D., Vipiana, F., Campione, S., & Campione, S. (2021). Symmetric Triangle Quadrature Rules for Arbitrary Functions [Conference Presenation]. https://doi.org/10.2172/1847207 Publication ID: 77313
-
Zinser, B.F., Blake, S., Pfeiffer, R.A., Huang, A., Himbele, J., Freno, B., Dang, V., Kotulski, J., Rajamanickam, S., Johnson, W., Campione, S., Langston, W., & Langston, W. (2021). Gemma: An Electromagnetic Code for Heterogeneous Computer Architectures [Presentation]. https://www.osti.gov/biblio/1847565 Publication ID: 77268
-
Sarma, R., Nookala, N., Reilly, K.J., Liu, S., De Ceglia, D., Carletti, L., Goldflam, M., Campione, S., Sapkota, K.R., Green, H., Wang, G., Klem, J.F., Sinclair, M.B., Belkin, M.A., Brener, I., & Brener, I. (2021). Strong Coupling in All-Dielectric Intersubband Polaritonic Metasurfaces. Nano Letters, 21(1), pp. 367-374. https://doi.org/10.1021/acs.nanolett.0c03744 Publication ID: 75130
-
Campione, S., Warne, L., Langston, W., Gutierrez, R., Hicks, J.W., Reines, I., Pfeiffer, R.A., Himbele, J., Williams, J., & Williams, J. (2021). Penetration through slots in cylindrical cavities with cavity modes overlapping with the first slot resonance. Electromagnetics, 41(2), pp. 98-109. https://doi.org/10.1080/02726343.2021.1879356 Publication ID: 75083
-
Jones, A., Campione, S., Stephens, J., Eckert, A., Warne, L., Huerta, J., Pfeiffer, R.A., & Pfeiffer, R.A. (2020). UNCERTAINTY QUANTIFICATION IN ELECTROMAGNETIC [Conference Presenation]. https://doi.org/10.2172/1837143 Publication ID: 72233
-
Guttromson, R., Lawton, C., Halligan, M., Huber, D., Flicker, J., Hoffman, M., Bowman, T., Campione, S., Clem, P., Fiero, A., Hansen, C., Llanes, R., Pfeiffer, R.A., Pierre, B., San Martin, L., Sanabria, D., Schiek, R., Slobodyan, O., Warne, L., & Warne, L. (2020). Electromagnetic Pulse – Resilient Electric Grid for National Security: Research Program Executive Summary. https://doi.org/10.2172/1879618 Publication ID: 71301
-
Campione, S., Warne, L., Langston, W., Pfeiffer, R.A., Martin, N., Williams, J., Gutierrez, R., Reines, I., Huerta, J., Dang, V., & Dang, V. (2020). Penetration through Slots in Cylindrical Cavities Operating at Fundamental Cavity Modes. IEEE Transactions on Electromagnetic Compatibility, 62(5), pp. 1980-1988. https://doi.org/10.1109/TEMC.2020.2977600 Publication ID: 72872
October 13, 2022