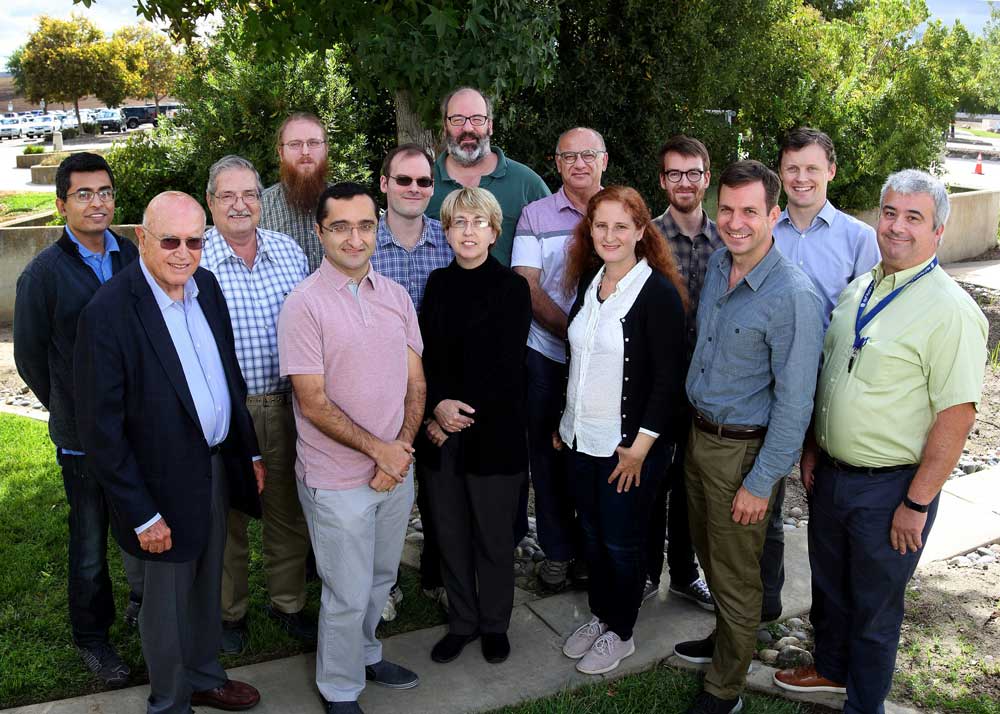
A successful partnership to help make aspects of chemistry research faster and more productive was recently renewed for another four years.
The Exascale Catalytic Chemistry project with Sandia, Argonne and Pacific Northwest national laboratories, as well as Brown and Northeastern universities, started in 2017 and brings together physical chemists and applied mathematicians to design computational tools to take advantage of the most powerful computers in the world to speed up understanding of heterogeneous catalysis, a complex chemistry problem.
Gas-phase molecules transformed on metal surfaces
Judit Zádor, the project director of Exascale Catalytic Chemistry, assembled the team of experts to develop models for heterogeneous catalysis — reactions of gas-phase molecules that take place on metal surfaces — faster and more reliably.
“What this project brings to catalysis research, is that it tries to automate the creation of complicated models that are necessary to describe the complex chemistry between gases and the catalytic surface,” Judit said. “Even for seemingly simple systems, like the hydrogenation of CO and CO2, there can be many dozens of reactions that take place on a simple facet of a metal. This can grow to hundreds or more if we consider larger molecules and more complex surfaces.”
Chemists and engineers actively study these interactions in problems including the conversion of simpler, cheaper molecules to more useful, expensive ones. With the new tools developed, Judit’s team at Sandia and beyond can create models and simulate these reactions more easily and systematically.
“People traditionally assemble these reaction mechanisms by trying to enumerate the relevant reactions manually the best they can, and then calculate the properties for each reaction individually. It’s a slow process and can be error prone,” Judit said.
“Our partners at Brown and Northeastern created a computer code that can enumerate the reactions and estimate their properties for you in a systematic way,” Judit continued. “At Sandia we then create codes to systematically, yet automatically, study these reactions using quantum chemistry. We also made simulation and analysis tools to interpret the models as a whole. Pacific Northwest National Laboratory contributes by its expertise in the underlying quantum chemistry method, while Brown, Argonne and Sandia jointly develop new methods to improve the thermochemistry.”
Improving chemistry one bit at a time
Besides uncovering interesting science about particular systems, an important goal of the project is to give other researchers tools that can more accurately predict their own systems of interest and eventually focus experimental efforts on the most productive catalytic strategies. These systematic computations can more accurately predict which interactions will lead to a desired chemical reaction.
Judit said that finding which interactions are most important to model is akin to knowing which branch of a tree to prune to take the shape you want.
“On a catalytic surface there are always chemical pathways that end up where you do want, but there are pathways that end up with a product you don’t want,” she said. “If you imagine the tree, you can follow one branch to the right, and it leads to the right outcome, but follow to the left, and it leads to an undesirable outcome. If you have an automated tool and enough computational power, you can examine many more scenarios than traditionally theoretically or experimentally possible and help you understand what makes a catalytic reaction produce a given product.”
A big reason why chemistry researchers need tools provided by high-performance computing is that there are so many possible reactions to measure or calculate.
“These days we can afford to do accurate computations not just for the top few most important reactions but for many more, and we get improved reaction rate estimates,” Judit said. “The strategy of this project is to improve the models iteratively. You propose a mechanism, you select the most important but least known parts, you improve them and then you plug it back into the original mechanism. Now you have a better mechanism, and if it’s still not good enough, you make another round. This circular improvement is a key concept of this project. If you go around enough times you ought to achieve your desired accuracy.”
The next phase
Now that the Exascale Catalytic Chemistry project — funded by DOE’s Office of Science, Basic Energy Sciences, Chemical Sciences, Geosciences and Biosciences Division — was renewed for another four years, Judit and her team want to study how the chemistry of a given molecule on a catalytic surface is altered by the presence of other molecules on a surface.
“These so-called co-adsorbates change the outcome of the reactions, so they are important. However, setting up calculations for these systems leads to extreme complexity, because there are just too many ways in which these molecules can interact on a surface.” Judit said. “You can’t do that by hand, and it seems that you can’t do that by sheer computer power only either. We will have to use machine learning to leverage our computational frameworks. It’s an exciting challenge.”
Read more about the Exascale Catalytic Chemistry project and its achievements.