Velocity-Mapped Ion Imaging hits 30 years of research at Sandia
Thirty years ago, David Chandler (8300) built an apparatus at Sandia’s Combustion Research Facility (CRF) to image the fragments of a molecule as it fell apart. At the time, David had no idea what a tremendous impact his research would have on understanding how molecules behave when excited by light or struck by another molecule or atom.
Since its first description in a 1987 publication coauthored by David and his collaborator, Prof. Paul Houston, this breakthrough research — first called photo-fragment imaging and now called, as the technique has evolved, velocity-mapped ion imaging (VMI) — has been referenced in almost 2,000 peer-reviewed, scientific articles. To celebrate the 30th anniversary of the VMI technique, the Journal of Chemical Physics has devoted a special issue in 2017 to VMI and has invited three guest editors: David, Houston, and Prof. David Parker of Radboud University.
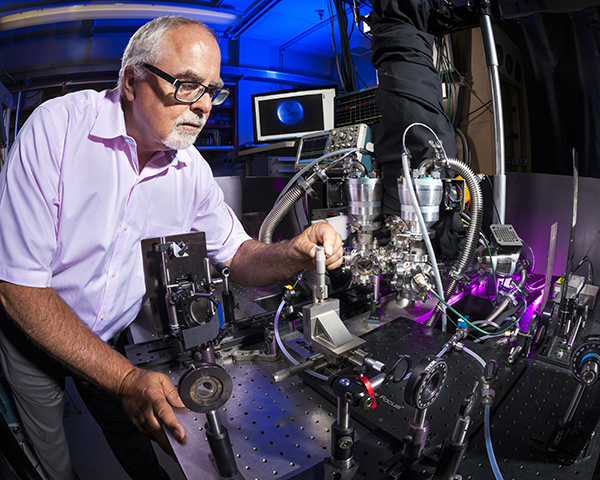
The VMI technique makes it possible to take a picture of a fragmenting or a colliding molecule at a specific time. The apparatus used in VMI is essentially a velocity microscope for gas phase charged particles (electrons, atomic, or molecular cations or anions). Analysis of the image provides the speed and direction — that is, the velocity — of slowly moving charged particles.
VMI can be used to study any process that generates a slowly moving charged particle. A more difficult problem was measuring the velocity of slowly moving neutral atoms and molecules. Shining ultraviolet light on most neutral particles converts them into charged particles without changing their velocity. So VMI can also be used to measure the velocity of a slow-moving neutral particle after their conversion to charged particles. This was the first application of the technique by David and Paul.
Before VMI, determining the velocity of a neutral molecule required a very high-resolution laser and many measurements to obtain the same information David now acquires with a single VMI image.
“The loss of an electron from a neutral molecule doesn’t change its velocity because the electron weighs so little,” David says. “It’s as if you were running down a street. If a dime fell out of your pocket, you wouldn’t suddenly accelerate. But if you had been carrying a bowling ball and dropped it, you would speed up.”
VMI used to study chemical processes
The VMI technique has been used to study many chemical processes, such as the dissociation and ionization of molecules excited by light. For example, photolytically ejecting an electron from a molecule produces a negatively charged electron and a positively charged atom or molecule. Each resulting charged particle’s velocity can be measured with the VMI technique to provide information about this process. The imaging of the electron is a version of photoelectron spectroscopy. VMI can also examine molecular collisions that change the molecules’ velocity and energy content or that directly create charged particles.
“With this technique we can determine the microscopic details of how molecules exchange energy when they collide,” David says. “This is a fundamental process that happens in reacting systems such as flames. You have to understand this energy transfer process extremely well if you want to understand and predict how reactions happen at high pressures.”
In addition to studying collisional energy transfer at the CRF, David has led CRF research efforts that focus on photochemistry and understanding how molecules dissociate — or fall apart — after being excited by light that has a greater energy than the energy of the molecule’s chemical bond.
VMI has provided insight into both the process by which molecules fall apart and the energy of the broken bonds. For instance, one Sandia study measured the energy of the C-H bond in acetylene, an important quantity needed for unlocking the acetylene combustion mechanism. David’s use of VMI at Sandia has enabled a greater understanding of processes that underlie combustion, providing critical information for developing accurate, science-based combustion models.
“We look at how much energy is transferred in a single collision and the directions in which the two molecules involved in the collision recoil,” David says. “Additionally, we use the polarization properties of lasers to determine how the molecule spins and vibrates as it recoils from the collision. Does a molecule spin like a Frisbee, act like a propeller, or turn a cartwheel as it bounces off of another molecule?”
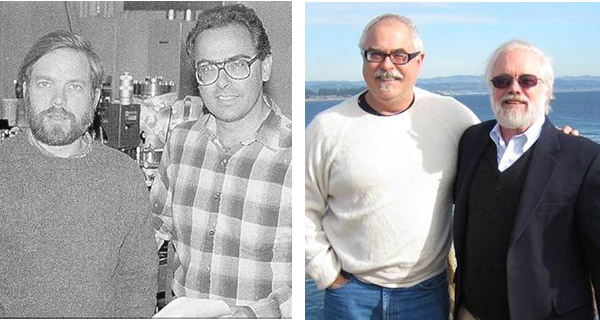
1980s: Research begins
When David began his career in photochemistry at Sandia/California in 1982, there was great interest in researching nitrogen oxide chemistry (NOx). During this period, his roommate and colleague, Robert Perry, found that adding the HNCO molecule to exhaust gases removed NOx pollutants from the process.
“Through photochemistry, we learned about the energetics of the bonding of the HNCO molecule. HNCO reacts with nitrogen oxides at pretty low temperatures to make water, carbon dioxide, and nitrogen,” says David. “Photochemistry allowed us to better understand the bonding within the HNCO molecule and therefore contributed to the understanding of how the molecule reacts to get rid of NOx pollutants.”
At a photochemistry meeting in 1986, David presented his HNCO work and heard a talk by Prof. Paul Houston, who was then at Cornell University. During the talk, it occurred to David that there was a better way of measuring the velocity of the products of photochemistry. David invited Paul to the CRF to conduct research on the photochemistry of the methyl iodide molecule. The first paper demonstrating the ion imaging technique in 1987 was a result of that collaboration.
After leaving Sandia, Paul performed, at Cornell, the first experiments that used ion imaging to study bimolecular collisions. He crossed a beam of NO molecules and a beam of argon atoms. He then observed how the NO molecules recoiled from the argon.
“It was like holding two water hoses spraying at each other, except they were gas hoses,” David says. “About 99 percent of the time, the molecules in one beam pass right through the other beam without colliding. But every once in a while, a collision occurs, resulting in some energy transfer.”
Those excited molecules are detected by ionization from an ultraviolet-colored laser beam and then imaged by an ion imaging apparatus.
Because laser beams are used to produce ionization of the neutral products, properties such as beam polarization and color are critical for understanding the process being studied. For instance, different colors of laser light can detect molecules that have varying amounts of rotation or vibration. A laser beam polarized in one way may detect molecules that are spinning clockwise, while a laser beam polarized in another way may identify counterclockwise-spinning molecules. Such measurements are of great interest to theoretical chemists who try to predict the behavior of atoms and molecules when they collide or are born in a photochemical process.
1990s: Improving the apparatus
“In the late 1990s, Dave Parker (Professor at UC Santa Cruz and later at Radboud University) worked with me at the CRF learning to conduct ion imaging,” says David. “After he returned to his university in the Netherlands, he made a really big improvement to the apparatus by adding a high-resolution electrostatic lens.” This higher resolution allowed researchers to measure the speed of charged particles with greater precision, thereby providing new information on processes that had previously been studied. It was after this improvement that the ion imaging technique became known as the Velocity-Mapped Ion Imaging technique.
Over the years, researchers have modified and improved ion imaging in various ways. One popular variation is the “slice-imaging” technique, pioneered by one of David’s ex-Sandia postdoctoral fellows, Theofanis Kitsopoulos. In this variation of the VMI technique, the detector is turned on and then off very quickly so that only a portion of the moving atoms or molecules is detected. This technique can simplify the analysis of some problems.
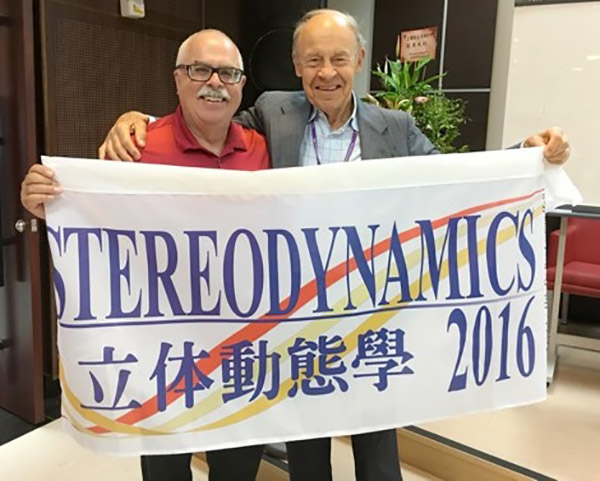
VMI today
Currently, David uses VMI to investigate the laser alignment of the H2 molecule with the strong electric fields associated with laser beams. He is also studying the collisional energy transfer of very vibrationally excited molecules. These molecules, which are excited by a laser beam to an energy where they are almost falling apart, dissociate after a single collision provides a small kick of extra energy. David is ionizing the fragments and measuring their velocity with the ion-imaging apparatus to learn about the energy kick.
The primary funding source for David’s VMI work is DOE’s Basic Energy Sciences office through the Gas Phase Chemical Physics program.