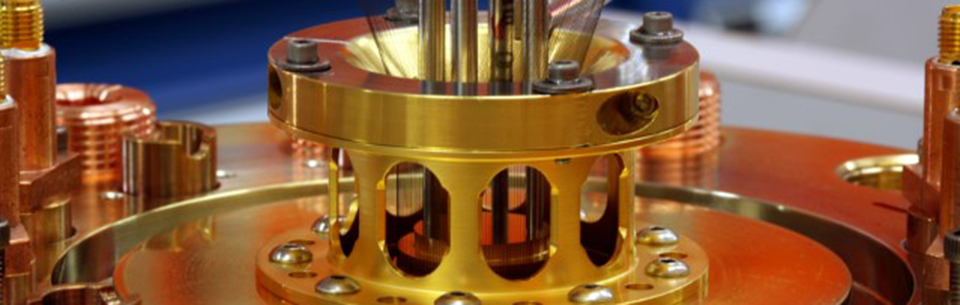
Astrophysics
Z Machine Experiments Address Important Astrophysical Questions
Sandia’s Z Machine provides us with the capability to delve deep into discovery science to study the behavior of matter at extreme conditions. This contributes to our fundamental understanding of nature. Most of the universe’s matter is under the extreme conditions that we study with Z. It is surprising to think that the conditions that we are accustomed to here on Earth are actually quite rare in the universe.
Why can’t we accurately model the structure of the Sun?
The evolution and internal structure of stars like our sun depend on how efficiently radiation created in the stellar core is transported outward to the surface. The material property that controls this radiation transport is called the “opacity.” Presently, predictions using the Standard Solar Model fail to capture the true location at which energy transfer becomes convective. This failure to match helioseismic observations of the boundary between the radiative and convective zones could possibly be explained by inaccurate opacities.
The predominant way to test opacity models is to measure the frequency resolved transmission of a bright x-ray backlight through a thin sample. The well-known requirements for plasma transmission measurements include production of uniform samples heated to the desired conditions, independent density increase because producing uniform plasma samples becomes more challenging and the x-ray backlight must be very bright. This has previously prevented laboratory opacity measurements at stellar interior conditions. Nature, a weekly journal of science, published Sandia’s article by Jim Bailey, et al. focusing on A higher-than-predicted measurement of iron opacity at solar interior temperatures.
Z recreates the temperature and density conditions inside the Sun to study the energy transfer efficiency in relevant materials. Shown below is a thin foil containing iron, an important stellar constituent, and then placed over the stellar interior conditions and heated to more than two million degrees.
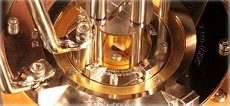
Experiments on Z can produce uniform, long-lived, and large plasmas with high volumes, temperatures, and electron densities. These unique characteristics and the ability to radiatively heat multiple experiments in a single shot, have led to a new effort; the Z Astrophysical Plasma Properties (ZAPP).
The focus of the ZAPP collaboration is to reproduce the radiation and material characteristics of astrophysical plasmas as closely as possible in the laboratory. This SNL research managed by Greg Rochau demonstrates that these opacity experiments have implications on the understanding of every Sun-like star in the universe. Future work focuses on refining these measurements and assessing the impact on the models of the Sun.
What are the characteristics of matter close to a black hole?
Most galaxies, like our Milky Way, are believed to host a black hole at the galactic center. Surrounding the center of some galaxies is a large “warm absorber” plasma heated by radiation emitted from the black hole accretion disk. Astronomers infer the warm absorber conditions by observing x-ray absorption and emission spectra with space-based observations. However, this requires a spectral synthesis model for photoionized plasma, a class of plasma impossible to study in the laboratory until the advent of high energy density facilities such as the Z Machine.
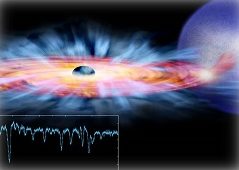
X-rays observed from black holes originate from atoms affected by extreme radiation. The intense x-rays on Z emulate the key radiative processes near a black hole and how they alter the electrons bound to the atoms. Experiments include utilizing silicon foil which is ionized by an x-ray flux of one trillion watts per square centimeter. Sandia’s scientists are studying the characteristics of this matter by conducting experiments that should enable an evaluation of models for spectral line formation in black hole accretion disks impacting our understanding for the physical picture of black hole disk structure.
How old are the stars in our Galaxy?
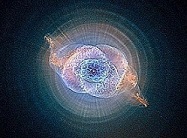
Recent observations call into question our understanding of the ages of White Dwarfs – the oldest stars in our Galaxy. White Dwarf stars are useful for a host of astronomical information including cosmochronology, which is the dating of stellar populations to constrain the age of the universe.
The Z Machine recreates the atmospheres of white dwarf stars so that we can study the effect of plasma conditions (age) on the emitted light.
This is a large (24 cm3) hydrogen gas cell heated to more than ten thousand degrees.
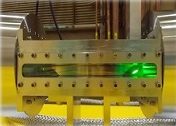