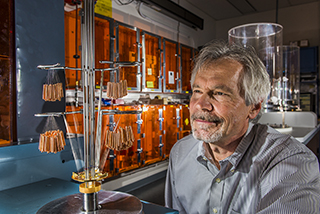
THE SUN NEVER LOOKED SO CLOSE — Physicist Jim Bailey observes a wire array that will heat foam to roughly 4 million degrees until it emits a burst of X-rays that heats a foil target to the interior conditions of the sun. Working at Sandia’s Z machine, Jim and his team have been able to determine experimentally, for the first time in history, iron’s role in inhibiting energy transmission from the center of the sun to near the edge of its radiative band. (Photo by Randy Montoya)
Working at temperatures matching the interior of the sun, researchers at Sandia’s Z machine have been able to determine experimentally, for the first time in history, iron’s role in inhibiting energy transmission from the center of the sun to near the edge of its radiative band.
Because that role is much greater than formerly surmised, the new, experimentally derived amount of iron’s opacity — essentially, its capacity for hindering the transport of radiative energy originating in nuclear fusion reactions deep in the sun’s interior — helps close a theoretical gap for a theory called the Standard Solar Model, widely used by astrophysicists as a foundation to model the behavior of stars.
“Our data, when inserted into the theoretical model, bring its predictions more closely into alignment with physical observations,” says lead investigator Jim Bailey (1683). His team’s work appeared Jan. 1 in the journal Nature.
The gap between the model and observations appeared in 2000 when analysis of spectra emerging from the sun lowered the estimated amount of energy-absorbing elements such as oxygen, nitrogen, and carbon by 30 to 50 percent. The decreased abundances meant that, plugged into the model, energy would arrive at the sun’s radiative edge more readily than before. This created a discrepancy between the star’s theoretical and measured structure. (Structure here refers to the sun’s varying temperatures and densities at different spatial locations.)
What was needed to reestablish the model agreement with observations was a way to balance the decrease in resistance to radiation transport caused by the lowered amounts of some elements.
Jim’s experimental group, including Taisuke Nagayama, Guillaume Loisel, and Greg Rochau (all 1683), in painstaking experiments spanning a 10-year period, discovered that the worldwide astrophysical estimate of the wavelength-dependent opacity of iron should be increased between 30 to 400 percent. The variation does not represent a large uncertainty but merely that iron’s opacity varies with the wavelength of the radiation.
“This represents roughly half the change in the mean opacity needed to resolve the solar problem, even though iron is only one of many elements that contribute,” the authors write in their paper.
One of the most mysterious places in the universe
Previous difficulties in getting accurate data has been that “the inside of a star is one of the most mysterious places in the universe,” Jim says. “It’s too opaque for distant instruments to see inside and analyze reactions within it, and too hot to send a probe into it. It has also been too difficult to run tests under appropriate conditions in a laboratory. So the physics that describes how atoms, embedded in solar plasma, absorb radiation, has never been experimentally tested. Yet that process dominates the way energy generated by nuclear reactions in the sun’s interior is transported to the outside.
“Fortunately, in our Z experiments, we can create temperature and density conditions nearly the same as the region inside the sun that affects the discrepancy the most — the edge of the zone where radiative energy transport dominates — in a sample that’s big enough, lasts long enough, and is uniform enough to test. We used that new capability to measure the opacity of iron, one of a few elements that plays the most important part in radiative energy transfer.”
Iron is important because it maintains the highest number of bound electrons that are essential in radiative energy transfer of any element abundant in the sun.
Still, the upward revision of opacities as a solution is bound to be controversial.
“No matter what we do, we can’t make measurements at all the different conditions we need to know,” team member Taisuke says. “There are 20 elements present, and a large range of temperatures and densities. We study iron because its complex electronic structure is a challenge to represent in opacity theories. And it is important in solar physics. The sun is a test bed to model other stars. Without experimental tests we don’t know if these models are accurate. To the extent we fail to understand the sun, then the workings of other stars are subject to some uncertainty.”
The target design of the experiments most recently involves intermingled iron and magnesium, tamped by plastic and beryllium layers on both sides. Radiation streaming through the sample heats up the iron and magnesium, which expand. The plastic restrains the expansion to keep it more uniform for opacity measurements. Magnesium provides information about corresponding density and temperature.
As for Jim, he often can be found by the business end of Sandia’s Z machine, which creates the temperature of the sun’s interior — about 2.1 million degrees —in a target about the size of a grain of sand.
From that small sample, Jim is able to do what theorists cannot: He can hold tangible evidence for the way iron atoms behave inside stars in his hand.
The work was sponsored by NNSA and the DOE Office of Science.