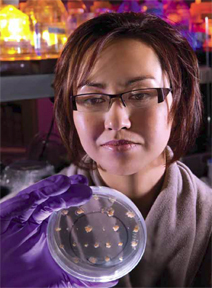
Sometime in the not-so-distant future, agricultural waste, weeds, and other plant products that are typically discarded or destroyed may fuel our cars. But going from the ground to the fueling station is not a simple process . . . yet. Sandia researchers are partnering with the US Department of Agriculture (USDA) on a project that aims to create a consolidated biorefinery process within plant cells.
Called “A Trojan Horse Strategy for Deconstruction of Biomass for Biofuels Production,” this project seeks to embed into the plant cells synthetic genetic circuits constructed using parts from extremophilic organisms that can break down the complex carbohydrates of plant biomass into fermentable sugars. The result would be a significant reduction in the cost and complexity of the process currently used to deconstruct biomass for biofuels processing — a savings of as much as $1 per gallon of biofuel by some estimates.
Masood Hadi (8621), principal investigator for the Trojan Horse project, explains that breaking down complex carbohydrates into biomass involves many physical processes — raising the temperature, subjecting the biomass to acid treatment followed by washing, and then enzymatic digestion.
“The pretreatment and enzymatic hydrolysis steps represent about 40 percent of the cost, which could be cut in half if the enzymes are embedded into dedicated biofuel crops,” he says. “Our idea is to consolidate this entire process and have it all take place within the plant. To put it simply, we’d move certain aspects of the biorefinery process inside the plant cells.”
The potential of the extremophile organisms is well-understood, thanks to Sandia’s research on using enzymes produced by Sulfolobus solfataricus as a means of breaking down lignocellulosic biomass (Lab News, June 22, 2007). But bringing organisms that exist only in harsh environments, such as sulfur cauldrons and hot springs, into living plants is not a natural fit.
Synthetic biology
This is where synthetic biology comes in: taking engineering design principles and applying them to the cellular structure of plants. “We’re taking a circuit that doesn’t necessarily ever exist in a tractable organism, putting it into a plant, and having that plant do something it couldn’t do before,” says Masood.
The goal is for the circuit to become part of the plant genome and be passed onto future generations through seeds. This is the Trojan Horse aspect to the project; the embedded circuits are, in a sense, stealthily brought into the plants where they lay dormant until activated by extreme heat.
Masood and his team are taking several approaches to the circuit construction — freely expressing the enzyme genes within the cell and targeting the genes to be near the complex carbohydrate material, minimizing mass transport and localization issues. Mary Bao Tran-Gyamfi (8621) and other Sandia microbiologists have developed constructs with different localization signals (kind of like zip codes) in different parts of the cell.
Two model plants
Initial work is being done on two model plants: Arabidopsis, a small flowering plant that is a member of the mustard family, and Brachypodium distachyon, a grass species related to the major cereal species (wheat, barley, oats, maize, rice, rye, sorghum, and millet). Using model systems in plants is akin to biological experiments with mice or fruit flies for translational research in humans: Model plants have well- understood genetics and are easy to transform, with small statures and short life cycles.
For help with the plant genetics side of the problem, Sandia is partnering with two scientists at the USDA’s Western Regional Research Center (WRRC) in Albany, Calif. — James Thomson, a bacteriologist and Arabidopsis expert, and Roger Thilmony, a molecular biologist and Brachypodium distachyon expert. The WRRC is home to the Brachypodium Genome Resources Project.
Thilmony and Thomson are now, through biotechnology tools, expressing these genes of interest, the engineered circuits, in the model plants. The process to grow plants with the genes will take five to six weeks with Arabidopsis and three to four months with Brachypodium.
Proof of concept
The USDA researchers already have successfully transformed onion cells as a proof of concept. Because onion cells are white and the genes are blue, it’s quickly apparent if the transformation took place and the circuit worked in the plant cell.
Getting the genes into the plants is an amazing process. For Arabidopsis, Thomson creates an agrobacterium, to which he feeds the DNA encoding the circuit. Then he literally dips the plant flowers into the bacteria solution and the bacteria take it from there.
“The bacteria serve as a shuttle vector that takes the DNA into the plant — it does the hard work. We just wait a few weeks to see if the plant seeds are transformed as we hope,” explains Thomson.
The process is more complicated with Brachypodium; the bacteria must be introduced to plant stem cells, but the general principle is the same.
Advanced imaging
Sandia is also drawing on its expertise in advanced imaging and analysis techniques developed by Jeri Timlin in Biomolecular Analysis and Imaging Dept. 8632. “Our techniques in hyperspectral fluorescence imaging with multivariate curve resolution enable researchers to remove the background of chlorophyll and locate the proteins of interest,” she says.
Her work, along with that of colleagues Michael Sinclair and Ryan Davis (both 1816), helps Masood and other researchers see results quickly and clearly. This information is then used to modify and enhance future experiments.
If the circuits are successfully introduced into the model plants, the process will be repeated with dedicated biofuel plants such as switch grass, which is closely related to Brachypodium, and poplar, a close relative of Arabidopsis. “If we are successful in Brachypodium, I’m quite confident we can express the genes in switchgrass,” says Thilmony.
In parallel, the researchers are also investigating rice straw as another potential dedicated biofuel. “About 50 percent of the world’s protein comes from rice, but rice straw is discarded and even burned,” says Blake Simmons (8655), manager of the energy systems group and the Trojan Horse project manager. “So we’d be making energy from something that typically goes to waste.”
Not competing with food crops
This is an important point — the biofuel crops do not compete with agricultural crops used for human or even animal consumption, thus avoiding the “food versus fuel” debate. Another important point is that the synthetic biology is not being done on food-source plants. In other words, these aren’t genetically engineered foods.
“With rice straw, this enzyme will not be expressed in the grain. It will only be in the stalks and leaves, which are not even used for livestock because the silica content is too high for any animal system consumption,” says Masood.