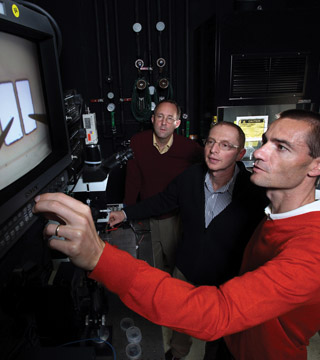
Sandia researchers have devised a novel way to realize electrical conductivity in metal-organic framework (MOF) materials, a development that could have profound implications for the future of electronics, sensors, energy conversion, and energy storage.
A paper to appear in Science magazine, “Tunable Electrical Conductivity in Metal-Organic Framework Thin-Film Devices,” debuted in the Dec. 5 edition of Science Express. The paper — co-authored by a group of Sandia researchers and collaborators at the National Institute of Standards and Technology (NIST) — describes a technique that experiments show successfully increases the electrical conductivity of one MOF by more than six orders of magnitude.
“Fundamentally, this sheds enormous light on the conduction process in these materials,” says material scientist Alec Talin (8656), the paper’s lead author.
Applications for electrically conducting MOFs, says senior scientist Mark Allendorf (8600), include chemical sensing, medical diagnostics, energy harvesting and storage, and microelectronics.
MOFs: ‘Tinker Toys’ for chemists
Materials researchers have considered MOF materials primarily for use in gas storage, drug delivery, and other conventional applications for porous materials. Their crystalline structure, which resembles molecular scaffolding, consists of rigid organic molecules linked together by metal ions.
This hybrid of inorganic and organic components produces an unusual combination of properties: nanoporosity, ultra-high surface areas, and remarkable thermal stability, which are attractive to chemists seeking novel materials that combine the superior performance of traditional inorganic semiconductors with the low cost and ease of fabrication typical of conducting organic polymers.
Mark, a chemist and MOF expert who called the research findings the most exciting development in his 28-year Sandia career, likens them to “tinker toys” for chemists.
“When you imagine the ‘tinker toys’ we played with as children, you recall they are essentially wooden balls with holes that you can link together with sticks,” Mark says. “MOFs work the same way, only you substitute metal ions for the balls and organic molecules for the sticks.”
The resulting open space within the scaffolding can be filled with guest molecules, which gave Alec the idea to use the pore to make the MOFs electrically conducting.
“Importantly, MOFs possess a characteristic of molecules that allows us to adapt their properties to a specific application. We can perform chemistry on them, unlike traditional inorganic electronic materials, such as silicon and copper,” says Alec. Molecules, he says, represent the “ultimate, small-scale unit” at which electronic devices can be made. They are so difficult to manipulate and organize, however, that practical “molecular electronics” have not been realized. “How you connect to molecules, where you place them — those issues have consistently perplexed materials scientists,” says Alec.
The power of empty space
So he considered a different approach. “With MOFs, we can get around this problem by using the nanopores to organize molecules. The trick is to pick the right kind of molecule, so that it binds to and interacts with the entire framework.” Some MOFs, says Alec, have empty holes in the tinker-toy balls that can bind molecules that infiltrate the pores.
“This isn’t like silicon, which can’t change its electrical properties,” Alec says. “You can add tiny amounts of dopants to silicon or introduce other impurities, but with our approach, you suddenly have the potential to tailor the material to achieve exactly the properties you want. This is the beauty of molecular electronics.”
To test their hypothesis, Sandia and NIST researchers added a molecule known as tetracyanoquinodimethane, or TCNQ, to their framework. First, they took a substrate with platinum electrodes patterned on its surface and coated it with a thin film of the MOF. The substrate was then dipped in a solution containing the TCNQ molecule, which they knew would seep into the MOF’s tiny pores. The MOF film containing the TCNQ bridged the electrode connection points, which then could be connected to a current meter for measuring.
“Frankly, I thought it would never work,” says Mark. “But that’s the great thing about science: Being wrong can be a good thing.”
The results are in, and they are good
The research team found that the MOF materials were conducting, though at relatively small quantities at first. “It was clear that something good was happening, so we were very excited,” says Mark.
The experiment was repeated several times with slight but important improvements in film quality achieved by optimizing the laboratory fabrication process.
“Conditions matter, and we had to be very deliberate in how we prepared the framework to accept the guest molecule,” says Mark. Removing the water and excess solvent from the film is no trivial matter, he says. The research team fine-tuned the process over the course of several months and, in doing so, began to see large leaps in electrical conductivity.
“The increase was massive,” says Alec. The conductivity in the material, he says, is now a million times higher than that of the starting material, and a thousand times higher than anything previously reported using a metal-organic framework.
The researchers plan to patent their approach and also hope to land additional funding to experiment with other guest molecules.
Keeping up with Moore’s Law and other applications
“The overwhelming success of this project opens a whole new way to design electrically active materials,” says Alec. Organic materials, he notes, offer low costs and mechanical flexibility. “There are probably hundreds of potential applications for this work that come into play, such as breath analysis and microelectronics,” he says.
The ability to make smaller and faster electronic devices to keep up with Moore’s Law has always been a motivator in the field of molecular electronics, Mark says. MOFs have the potential to create molecular electronic devices on the scale of their pore dimensions, or approximately 1 nm.
Solar technology is another potential application, says Mark, and DOE’s SunShot Initiative has funded some of the initial research. “With electrically conducting MOFs, we might very well be able to combine the high efficiencies achievable with traditional inorganic thin film materials such as polycrystalline Si with the low cost and flexibility aspects of organic photovoltaics,” he says.
“Our next step needs to be the exploration of other hosts and guest molecules,” says Alec. “We’d like to experiment with different MOF structures and different organic molecules to see if new behavior emerges. We want to see where this new learning takes us.”