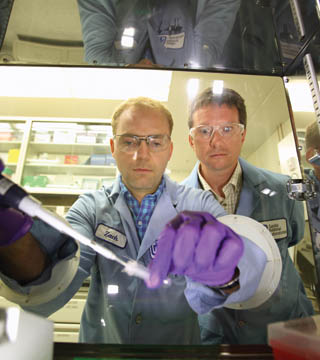
The original aim of the RapTOR (Rapid Threat Organism Recognition) Grand Challenge was to identify “unknown unknowns” — dangerous, virulent pathogens of unknown origin. Zach Bent (8623) and Steve Branda (8621) have now applied molecular biology capture approaches developed by the RapTOR team to a different problem, understanding how known pathogens become virulent. Until recently, determining what made pathogens dangerous was too expensive and time-consuming, but the capture methods overcome that barrier and could help researchers develop diagnostics and therapeutics to better combat drug resistant pathogens.
Analyzing pathogens’ gene expression during infection has been difficult because pathogen RNA molecules (“signal”) are generally outnumbered by host-derived RNA (“background”) in the sample (e.g., infected blood) by about 100-fold. RapTOR began to address this signal-to-background ratio problem with a negative capture method that
uses affinity probes to capture and discard nucleic acids not of interest (e.g., host-derived) prior to sample analysis via Second Generation Sequencing (SGS). To amplify the pathogen signal, Zach turned to capture probes that selectively bind and recover pathogen nucleic acids for sequencing, a strategy referred to as pathogen capture.
“Our approach is very minimalist. It’s fast and cheap, which is important because it requires a large excess of probe, about 100 times more probe than sample,” says Steve. “We finely chop and tag pathogen nucleic acids to generate the probes. It’s crude, but it gets the job done. This method uses the entire genome, so every possible transcript can be captured and sequenced.”
A simple concept
The concept is simple: Saturate your sample with pathogen-specific probes; give the probes every opportunity to find their complementary nucleic acid partners; recover the hybridized probes on a column; wash extensively to disrupt non-specific interactions; and release the pathogen nucleic acids from the column for sequence analysis.
“It sounds straightforward, but carrying it out is tricky because the conditions have to be exact for the probe to find the target, grab it, and remain stable enough to isolate it from the sample,” says Steve. “Efficiently separating the target from the probe is key as well. You don’t want to waste time and money inadvertently sequencing the probe.”
Another challenge is making the probe discriminating enough to grab only nucleic acids from the pathogen, avoiding those from the host. This increases the degree of pathogen enrichment, which is critical to getting sufficient sequence coverage of the pathogen’s entire transcriptome at a reasonable cost. Zach and Steve’s goal was at least 100-fold enrichment.
Getting it right took about two years of painstaking research; Zach and Steve are grateful to the many interns and technologists who have helped on the project. Most improvements were incremental, but their breakthrough came with precise definition of the column washing conditions.
“We significantly raised the ionic strength of the washing buffer to increase the stringency of the wash. Suddenly almost all of the nonspecific binding we were getting went away. That brought our enrichment up from 10-fold to over 100-fold in a single step,” says Zach.
Seeing pathogens in high fidelity
Zach and Steve use pathogen capture in tandem with other molecular biology methods developed for the RapTOR project, and the results are quite amazing — the ability to see details of what the pathogen is doing, due to the sheer number and variety of pathogen gene transcripts that can be sequenced.
Zach puts the advance in perspective. “I spent all of my time in graduate school looking at the in vivo expression of four different genes. This required an intense amount of work to get results of any significance,” he says. “With our new technique, instead of looking at a few genes, I can look at expression of all of the pathogen’s genes, several thousand of them, at once.”
This technique mitigates a significant barrier to research — the cost of sequencing. While sequencing remains a fixed cost, the cost of prepping the sample is small (about $20 to prepare and capture a sample), and the sample itself is rich in genetic material. By enriching the sample for transcripts of interest, the researchers can load multiple samples on the sequencer and still get appropriate coverage depth. This yields better bang for the buck, in terms of sequencing costs, and also saves time because multiple samples are analyzed in a single sequencing run.
Zach and Steve tested the efficacy of their pathogen capture technique by analyzing in vitro infections. In a paper titled “Enriching pathogen transcripts from infected samples: A capture-based approach to enhanced host-pathogen RNA sequencing”, published in Analytical Biochemistry in March 2013, they reported 10- to 100-fold enrichment of reads mapping to the pathogen’s transcriptome, relative to untreated controls. This enrichment greatly increased the diversity of pathogen transcripts sequenced, as well as the coverage depth at which each transcript was sequenced.
They then closely studied F. tularensis at two critical transitions during infection: when the pathogen escapes from an internal membrane-bound compartment within the host cell, and when the pathogen begins replicating within the cytosol of the host cell.
“We saw what we expected: Two distinct gene expression profiles at those two transitions,” says Steve. Their work also revealed several hundred transcripts of unknown function, many of which are up-regulated (switched on) and therefore potentially important for pathogen survival and proliferation within the host cell. These results will be reported in a PLoS ONE article that is currently in press.
“We don’t have the resources right now to study those particular unknown genes,” says Zach. “Just the number of unknown genes we found is quite interesting, because Francisella has been studied extensively for two decades. The number of unknown, up-regulated genes we found in just this initial experiment shows that we really haven’t been seeing the whole picture.”
Understanding host/pathogen wargames
Moving forward, the researchers would like to study infections in even greater detail. Zach likens host/pathogen interactions to an arms race on a microscopic level. “The pathogen invades, the host responds, the pathogen counterattacks, and this goes on until one side wins,” he says. “Being able to look so closely at expression of all of the host and pathogen genes will let us figure out those key critical moments when the host is overpowered and an infection spreads.”
They want to expand their research to include animal studies to track the dynamics of infection at different physical locations, a particularly under-studied dimension of infection. The speed and low cost of the pathogen capture-based technique would enable tracking of an infection as it spreads to different parts of the body.
“By being able to sample many different physical locations, you can see how different body defenses are mounting a reaction, and what the pathogen is doing to survive,” says Steve. “Those have been really difficult experiments to carry out, and we don’t at all understand what it means for bacteria to survive in the liver versus a lung, for example. This is a completely different facet on the whole interaction between a pathogen and a host.”
In the future, pathogen capture could enable researchers to study bacterial gene function during infection with such precision that they can develop new countermeasures that are effective against drug-resistant strains. Therapeutics could be made to target specific bacterial pathways and infection mechanisms entirely separate from those targeted by broad-spectrum antibiotics. By hitting multiple pathways at once, says Steve, a therapeutic could conceivably stop a pathogen in its tracks, preventing it from developing resistance to the new drugs.
Zach is also the principal investigator of an Exploratory Express LDRD to analyze the Yersinia enterocolitica transcriptome at very early time points in infection using pathogen capture technology. “We aren’t quite finished analyzing all of the data, but we have already made several exciting discoveries that shed light on the roles of its various virulence mechanisms,” says Zach.
In October, Zach and Steve will begin working on an LDRD-funded project led by Robert Meagher (8621) to build an automated device to perform pathogen capture on 96 samples simultaneously. “Right now, we have a very specialized setup and it still relies on a lot of manual labor,” says Zach. “We want this to be accessible and compatible with a typical lab setup, so that other groups can take advantage of our technique.”
Developing the automated device will take a year or so, but during that time Zach and Steve will conduct more studies to prove the technology. “With each new development, you can do more experiments than you could in the past, and different types of experiments as well,” says Steve. “It’s been a long time in the making, and there’s still much more work to be done, but we’ll continue to get exciting results along the way.”