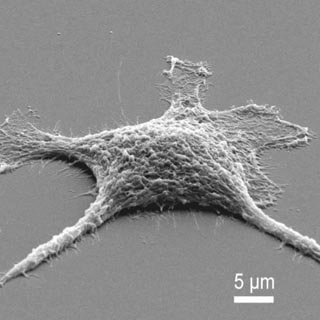
Sandia researchers have created “zombie” mammalian cells that may function better after they die.
The simple technique uses a silica solution to coat a cell’s insides to form a near-perfect replica of its internal structure. The process opens the door to simplifying a wide variety of commercial fabrication processes from the nano- to macroscale.
The work, reported in a fall issue of the Proceedings of the National Academy of Sciences (PNAS), uses the nanoscopic organelles and other tiny components of mammalian cells as fragile templates on which to deposit silica. The researchers then heat the cell to burn off its protein. The resultant hardened silica structures are faithful to the exterior and interior features of the formerly living cell, can survive greater pressures and
temperatures than flesh ever could, and will function better for some uses than when they were alive, says lead researcher Bryan Kaehr (1815).
Letting nature do the work
“It’s very challenging for researchers to build structures at the nanometer scale,” says Bryan, who came to Sandia as a Truman Fellow. "We can make particles and wires, but 3-D arbitrary structures haven’t been achieved yet. With this technique, we don’t need to build those structures — nature does it for us. We only need to find cells that possess the machinery we want and copy it using our technique. And, using chemistry or surface patterning, we can program a group of cells to form whatever shape seems desirable.”
Says University of New Mexico professor and Sandia Fellow Jeff Brinker (1002), “The process faithfully replicates features from the nanoscale to macroscale in a robust, three-dimensionally stable form that resists shrinkage even upon heating to over 500C. The refractoriness of these delicate structures is amazing.”
Because a cell is populated by a vast range of proteins, lipids, and scaffolding, says Bryan, its interior is ready-made to serve as models for catalysts, funnels, absorbents, and other useful nanomachinery.
For example, he says, “Catalysts that evolve in cells are large molecules (enzymes) that have to be in the right shape for their chemistry to work. Because structure is important to their function, if we can stabilize a catalyst in the shape it evolved, that’s extremely valuable. Imagine stabilizing a genetically designed biocatalyst at its most optimal shape and then using it in a 200 degree Celsius reaction in which it otherwise would have no chance of surviving.” The hardened silica would stabilize and protect the still-present protein as it did its work.
In its simplest, most immediate use, says UNM post-doctoral student Jason Townson, silicification may be the simplest, best method of preserving the structure of organic materials for imaging. “Formerly, for internal preservation and subsequent imaging, a cell would be fixed in formaldehyde or some other preservative. But many of these methods are labor-intensive. This method is simple. The preserved cells will never get sloppy in decay. And when we cracked open the resulting structure, we were blown away by how well the cell was preserved, down to the minor groove of the cell’s DNA.”
Like a Madame Tussauds wax portrait
Heating the cell to still higher temperatures, greater than 400 C, evaporates the organic material of the cell — its protein — and leaves the silica in a kind of three-dimensional Madame Tussauds wax portrait of a formerly living being. The difference is that instead of modeling the face, say, of a famous criminal, the hardened silica-based cells display internal mineralized structures with intricate features ranging from nano- to millimeter-length scales.
Construction occurs like this: Take some free-floating mammalian cells, put them in a Petri dish, and add silicic acid.
Through the action of methanol, a byproduct of the acid, the cell’s lipid layers — the protective casings that keep the cell intact — are softened and made porous enough for the silica to flow in at about the temperature of the human body.
The silicic acid, for reasons still partially obscure, enters without clogging and in effect embalms every organelle in the cell from the micro- to the nanometer scale.
If the cell isn’t heated, the silica forms a kind of permeable armor around the protein of the living cell. This may support it enough to act as a catalyst at temperatures and pressures undreamed of by nature.
“Once we’ve stabilized the cellular structure, it can still carry out reactions and more important, that reaction is stable enough to work at high temperatures,” Bryan says. “It’s a means to take a soft, potentially valuable biological material and convert it to a fossil that will stay on our shelves indefinitely.”
Ordinarily, preserving something organic means freezing it, which is energy-intensive, he says. Instead, “We’re doing rapid fossilization: quickly converting a protoplasmic cell into a hard structure that will stand the test of time.”
The unusual but simple procedure may serve as a model for creating hardier classes of nanoscopic products.
Experiments showed the cell can be used as a reverse mold from which, at 900 C, a porous carbonized structure results from heating cell protein in a vacuum. (Put in ordinary terms, burning wood in air leaves a residue of structureless soot. The zombie heating method results in a high-quality carbon structure.) Subsequent dissolution of the underlying silica support decreased the cell’s electrical resistance by approximately 20 times. Such materials would have substantial utility in fuel cells, decontamination, and sensor technologies.
Going beyond large sponges
That such extraordinary results can be achieved by silicifying cells indicates, according to the technical paper, that many soft cellular architectures could be “feedstock for most materials processing procedures, including those requiring high temperatures and pressures.”
Other porous material structures, relying on titanium instead of silica, have been formed using the organic template technique. Other metal oxides, says Bryan, are a possibility. These would have advanced structural functions or could serve as catalysts.
The work follows the efforts of a number of scientific groups, including Bryan’s, that have built gel-like structures, copied them with silica, and then burnt off the gel to create, in effect, large sponges.
“Now we can change the biological shape and calcify (heat) it, so for the first time we get new irregular structures,” Bryan says.
In the PNAS paper, Bryan’s team used dissociated cell culture from various human, chicken, and mouse organs as starter material. Grown in flasks, these are soft, delicate objects that normally would eventually exist in a multicellular structure like a person (or a chicken or mouse).
“Since then we have found that the procedure can silicify an entire organism — in this case, a chicken embryo — which is somehow creepy,” says Bryan. “But it proves that larger-order animal forms (that is, humans) can, in principle, be glassified just like single cells using this technique.”
The work was supported by DOE’s Office of Science. Other authors of the technical paper are Jeff Brinker (1002 and UNM), Brian Swartzentruber (1131 and the Center for Integrated Nanotechnologies), Robin Kalinich (2501), and Darren Dunphy and student Yasmine Awad of the University of New Mexico.