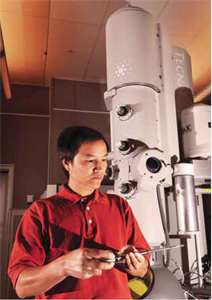
The very word diamond coaxes ideas of beauty and romance to the mind. Yet the gem is a carbon cousin to the relatively unlovely graphite we find in pencil leads, brake linings, and lubricants. Diamond is very hard and brittle, a good conductor of heat, and an electrical insulator; graphite is fairly soft, a poor conductor of heat, and electrical conductor. Put the two minerals under a microscope, and you’ll find they are made up of differently shaped crystals.
Diamonds are easily as mysterious as they are beautiful. Though it has colorless tetrahedral crystals, like stretched-out cubes, its carbon sister graphite has more complex, hexagonal crystals. Nature requires that crystals are formed when solid structures are formed from liquid. How then can carbon, which has never been reported in liquid form, become a diamond? Apparently the transformation occurs very quickly. This magic of mineralogy has now been replicated and witnessed in the laboratory — at Sandia, by research scientist Jianyu Huang.
Natural diamonds form deep below the Earth’s surface, when extremes of heat and temperature melt concentrations of carbon-bearing materials into a new material capable of being cut and polished into brilliant gemstone. Synthetic diamonds are made when huge presses provide the necessary heat and pressure to force carbon into a new form. Jianyu made his — momentarily — with a nanoscale heater. The big news is that, thanks to transmission electron microscopy (TEM), Jianyu was able to make a stop-motion record of “quasimolten” diamond as it took form and solidified again. Under normal atmospheric pressure and very high temperatures, diamond does not melt but instead turns into graphite.
Jianyu is quick to point out that he did not melt graphite with his experiments; nor did he produce liquid diamond. Nothing actually flowed — the process happened too quickly for this, before changing its state again. The material “flickers” between the two forms of carbon, melting then refreezing to its original state within a few seconds. This is called quasimelting, because no liquid flow occurs.
Jianyu’s discovery — made originally at Boston College, then analyzed and replicated at Sandia — offers a new method of studying the structures of carbon at extreme conditions in situ and at an atomic scale. On the geologic scale, such studies may increase understanding of the conditions that exist in Earth’s mantle, where natural diamonds form. The behavior of materials under extreme conditions of high pressure and temperature has long been of interest to researchers in physics, astronomy, and geology. Since diamond possesses the highest hardness, thermal conductivity, and melting temperature of all materials, it has been the focus of numerous studies at extreme conditions.
The Sandia experiments focused on heating tiny graphite spheres which, because they consist of many concentric layers of carbon, were dubbed “onions” by researchers Florian Banhart of the Institute for Physical Chemistry in Mainz, Germany, and Pulickel Ajayan of Rensselaer Polytechnic Institute, New York. In a research paper published in Nature in 1996, Banhart and Ajayan reported that they had changed diamond into graphite under conditions of high pressure and temperature. But they were unable to observe and record the process of transformation.
“They were able to convert ‘onion’ to diamond at about 700 degrees C under electron beam irradiation, using a TEM heating stage,” explains Jianyu. “We are heating only very locally, to a much higher temperature, using a carbon nanotube. That is why we observed the quasimelting behavior, whereas the 1996 experiment was unable to detect this process.”
Jianyu says this 1996 paper inspired him to apply nanotechnology to learn more about the quasimelting process. “While pursuing research in carbon nanotubes, I found that I could generate a temperature higher than 2,000 degrees C by passing a high current through the nanotube, similar to passing a current through a light bulb,” he says. “Then I thought, what will happen if I heat the carbon onion with my carbon nanotube? I thought it an interesting experiment because nobody had been able to generate such a high pressure and high temperature in situ before.”
In the Sandia experiments, the graphite-like onion, about 20 nanometers in diameter, is first bound to the nanotubes. By heating the carbon onions with the nanotube and simultaneously applying electron beam irradiation, temperatures inside the onion are raised to greater than 2,000 degrees C. The onion then self-compresses, generating very high internal pressure. “The pressure in the center of the onion is estimated to be over 400,000 atmospheres,” says Jianyu. “Isn’t that amazing!”
Sequential transmission electron microscope images made at the rate of two to three frames per second show in real time how diamond forms, then repeatedly melts and refreezes in crystal configurations that differ in terms of size, shape, internal structure, and crystal orientation. The structural changes in structure are determined by analyzing the changing image of the crystal lattice.
Jianyu now has Laboratory Directed Research and Development funding to investigate the thermal property of nanotubes, a remarkable material in its own right, with a host of special properties. But molten diamond will not be forgotten. “We’re still continuing the study,” he says. “We would like to know how the giant onion structure shrinks, how the shrink generates such high pressure, and how the graphite is initially converted to diamond. There is still a lot to explore.”