Big things often come in small — or in this case, thin — packages. Graphene, a single-atom-thick planar sheet of carbon atoms, is considered by many to be the next, big thing in nanoelectronics, the material that might keep Moore’s law in existence.
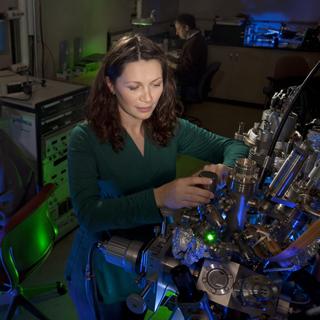
Graphene came into vogue about five years ago when two Manchester University scientists, Andre Geim and Kostya Novoselov, extracted it from bulk graphite. Graphene is chemically simple but physically strong and highly conductive. Electrons move through it at 1/300th the speed of light, significantly faster than through silicon. Scientists are excited by its potential for improved solar cells, LCD displays, touch screens, and other technologies.
Sandia researchers Elena Starodub, Kevin McCarty (far left) and Norm Bartelt (far right) around the Low Energy Electron Microscope used to study graphene growth. (Photo by Randy Wong) (Click on image to enlarge)
Norm Bartelt, Elena Starodub, and Kevin McCarty (all 8656) have been studying the formation of graphene for the past three years. This is no small task; graphene grows at extremely high temperatures, so its formation cannot be imaged using standard microscopy methods.
“There was a realization that you can do more with this material,” explains Norm. “We want to understand how it forms. With that knowledge, you can control growth to make better-performing materials.”
Recently, the team had a double breakthrough. They developed a method to image graphene in the earliest stages of formation and then they saw something very unexpected. It takes clusters of five carbon atoms to add area to a growing graphene sheet, an observation that greatly surprised Norm, a theoretical physicist.
“The rate at which carbon atoms attach should be proportional to the number of carbon atoms present,” he explains. “I’ve spent 20 years developing theories to explain how thin films grow, and none has behaved like this. We discovered that carbon atoms go through a complicated contortion before attaching to a graphene sheet.” To convince Norm, Elena repeated her measurements more than once, looking for an error that could explain the unexpected result.
Kevin developed the use of electron reflectivity to measure the number of carbon atoms on the surface. “I tried out the method on simpler systems, metal films growing on metal, and thought that it might work with graphene growth,” he says. “We found that the reflectivity is sensitive to minuscule amounts of carbon on the substrate surface.”
With practice, Kevin was able to image the growth of graphene while measuring the concentration of the carbon atoms floating around the surface — in a sort of pre-graphene configuration. “Once Kevin had learned how to measure very small concentrations of atoms attached to the surface, we then found this bizarre behavior of graphene growth, which was like nothing any of us had seen before,” says Norm. “We used information from the images that is usually ignored.”
Elena has been perfecting the technique of growing individual graphene crystals to continue studying how they develop. By closely monitoring the concentration of carbon, she can make what Norm describes as “tremendously large, perfect crystals.” This has led to more discoveries about the different phases of graphene on specific surfaces; for example, on the metal iridium the graphene crystals grow in four different orientations.
Peter Feibelman (1130) is doing first-principles calculations of the atomic structure of carbon and graphene on metal surfaces to help the team get a better sense of what is behind its behavior as it nucleates and grows. “Our method does not have atomic resolution, so we are guessing about where individual carbon atoms are,” says Norm. “With his first-principles calculations, Peter can develop hypotheses to test against our measurements.”
Peter’s contribution both validates and enhances the experimental work. “It gives us a much more complete description,” says Kevin. “We can then make statements about the behavior of individual carbon atoms.”
The project has generated six papers over the past 18 months, published in the New Journal of Physics, Physical Review B, and Carbon. The two articles in the New Journal of Physics were featured on the publisher’s “Select” website while the second paper in Physical Review B, “Graphene Growth by Metal Etching on Ru(0001),” was an editor’s selection.
The technique development began under a Laboratory Directed Research and Development (LDRD) project. The research is currently supported by the Office of Basic Energy Sciences and by an LDRD project to enable graphene nanoelectronics. “Using the knowledge developed through this research, graphene with extremely low defect densities is being grown and tested,” explains Kevin. “This is important because the material’s superb electronic and mechanical properties are only realized in samples of high crystalline quality.”