Links
Transportation Energy research at Sandia National Laboratories
Hydrogen and Fuel Cell Program at Sandia National Laboratories
Hydrogen Storage research at Sandia National Laboratories
Sandia’s Nanoelectronics and Nanophotonics Group
Hydrogen Materials Advanced Research Consortium
Hydrogen storage in metal hydrides
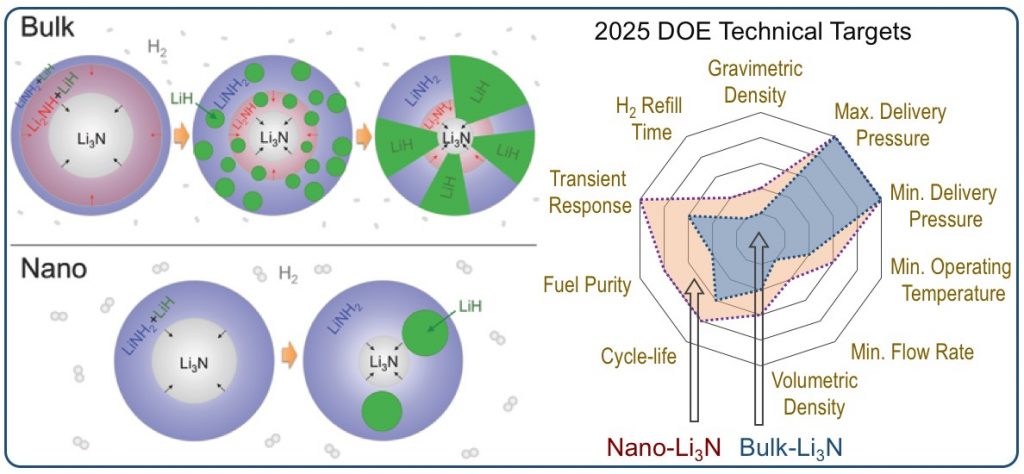
Hydrogen provides multiple advantages as an energy carrier, particularly when used in hydrogen polymer electrolyte membrane fuel cells. It is non-toxic, produces zero emissions at the point of use, and enables high efficiency conversion devices such as hydrogen fuel cells. Metal hydrides are promising solid-state hydrogen storage media, with record-high gravimetric and volumetric hydrogen densities. One of the most elegant and promising storage methods is through reversible metal hydrides, which can release hydrogen endothermically upon mild heating and absorb it exothermically when pressurized with hydrogen gas. Our research in this area is geared towards developing a foundational understanding of the phenomena that govern hydrogen uptake and release in metal hydrides. In particular, we are interested in understanding the effects of nanointerfaces that develop in materials upon cycling, and how they affect the macroscopic material properties.
- Schneemann, A.; White, J. L.; Kang, SY.; Jeong, S.; Wan, L. F.; Cho, E. S.; Heo, T. W.; Prendergast, D.; Urban, J. J.; Wood, B. C.; Allendorf, M. D.; Stavila, V. Chemical Reviews, 2018, 118, 10775-10839. ”Nanostructured Metal Hydrides for Hydrogen Storage”
- Carr, C. L.; Jayawardana, W.; Zou, H. Y.; White, J. L.; El Gabaly, F.; Conradi, M. S.; Stavila, V.; Allendorf, M. D.; Majzoub, E. H. Chemistry of Materials 2018, 30, 2930-2938. ”Anomalous H2 Desorption Rate of NaAlH4 Confined in Nitrogen-Doped Nanoporous Carbon Frameworks”
- Wood, B. C.; Stavila, V.; Poonyayant, N.; Heo, T. W.; Ray, K. G.; Klebanoff, L. E.; Udovic, T. J.; Lee, J. R. I.; Angboonpong, N.; Sugar, J. D.; Pakawatpanurut, P., Advanced Materials Interfaces 2017, 4, 1300803. “Nanointerface-Driven Reversible Hydrogen Storage in the Nanoconfined Li-N-H System”
- Stavila, L.E. Klebanoff, US Patent # 10000377 granted on 06/19/2018 (2018). “Nanostructured Metal Amides and Nitrides for Hydrogen Storage”
Metal-Organic Frameworks for device applications
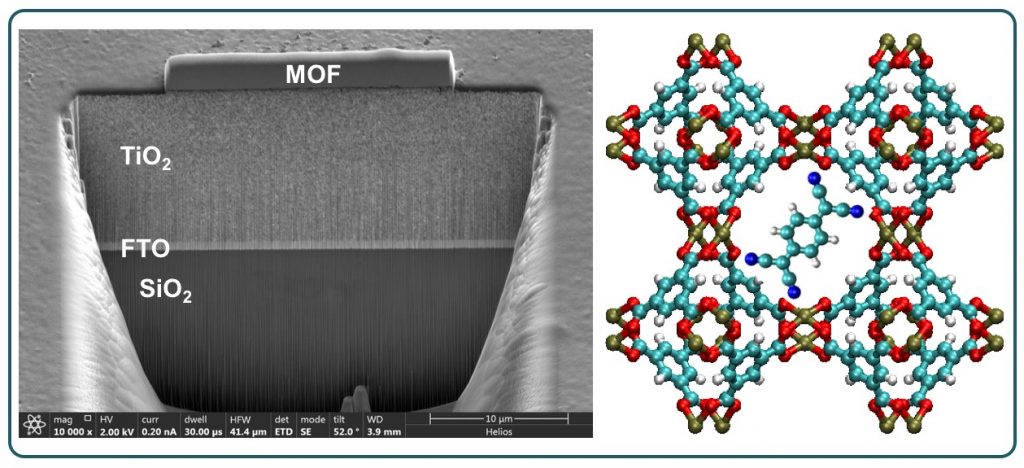
Metal-Organic Frameworks (MOFs) are normally thought of as insulating materials, however, with judicious choice of metal ion, linker and guest molecules, the MOFs can be rendered electronically conductive, so that appreciable amounts of charge can occur. The phenomenon is crucial to the implementation of MOFs in optoelectronic devices and sensors. Through various collaborations, we made contributions to the tool-box of techniques necessary to integrate MOF crystals and thin films with functional devices.
- Timothy C. Wang, Patrick F. Doty, Annabelle Benin, Joshua D. Sugar, Vitalie Stavila, Mark D. Allendorf, Chemical Communications, 2019, 55, 4647-4650. “Get the light out: nanoscaling MOFs for luminescence sensing and optical applications.”
- V. Stavila, A.A. Talin, M.D. Allendorf, Chemical Society Reviews, 2014, 43, 5994-6010. “MOF-based electronic and opto-electronic devices.”
- A.A. Talin, A. Centrone, A.C. Ford, M.E. Foster, V. Stavila, P. Haney, R.A. Kinney, V. Szalai, F. El Gabaly, H.P. Yoon, F. Leonard, M.D. Allendorf, Science, 2014, 343, 66–69. “Tunable electrical conductivity in Metal-Organic Framework thin-film devices.”
- D. Allendorf, A.A. Talin, F. Leonard, V. Stavila, US Patent # 9711743 granted on 07/18/2018 (2018). “Reconfigurable Electronics Using Conducting Metal-Organic Frameworks.”
Single-Site Catalysis
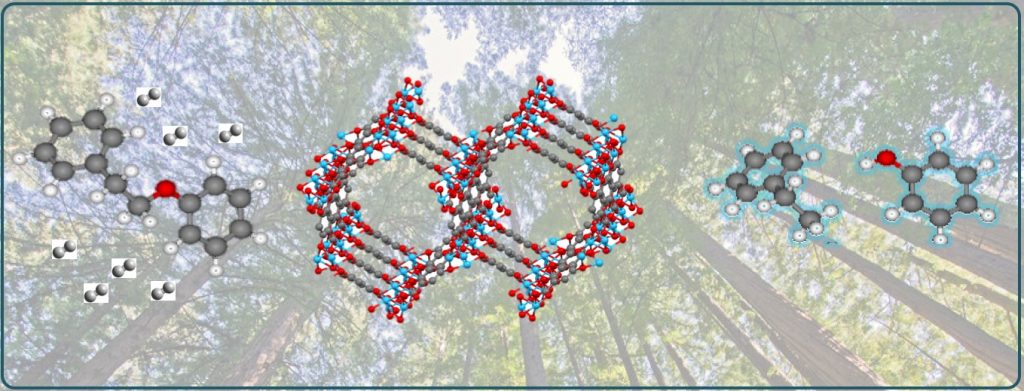
Our approach to the design of environmentally friendly and highly active single-site catalysts is based on principles of solid-state chemistry, biocatalysis, and computational chemistry. This enables rational design of novel open framework catalysts (such as MOFs and COFs) with the ability to independently control the concentrations of Lewis and Brønsted acid sites, as well as the pore size for size-selective catalysis. Single-atom catalytic sites within the open framework can stabilize reactive intermediates and prevent side reactions and deactivation. In this way we have succeeded to design a range of multifunctional catalysts, such as highly selective catalysts for C-O bond hydrogenolysis. Such catalysts could enable efficient processes for transformation of biomass into fuels and value-added products.
- Vitalie Stavila, Michael E. Foster, Jonathan W. Brown, Ryan W. Davis, Jane Edgington, Annabelle I. Benin, Ryan A. Zarkesh, Ramakrishnan Parthasarathi, David W. Hoyt, Eric D. Walter, Amity Andersen, Nancy M. Washton, Andrew S. Lipton, Mark D. Allendorf, Chemical Science, 2019, DOI: 10.1039/C9SC01018A. “IRMOF-74(n)–Mg: a novel catalyst series for hydrogen activation and hydrogenolysis of C–O bonds.”
- V. Stavila, R. Parthasarathi, R.W. Davis, F. El Gabaly, K.L. Sale, B.A. Simmons, S. Singh, M.D. Allendorf, ACS Catalysis, 2016, 6, 55-59. “MOF-Based Catalysts for Selective Hydrogenolysis of Carbon–Oxygen Ether Bonds.”
Ion transport in solid electrolytes
The majority of known solids are poor ionic conductors due to high activation energies and the lack of vacant sites for ion migration. Therefore, for a long time the ionic conductivity of solids has been insufficient to supplant liquid electrolytes in battery applications. Very recently, however, a number solids with ionic conductivities comparable to that of liquids have been discovered. The discovery of these fast ion conductors has advanced the prospects for realizing solid-state batteries with improved safety, voltage and energy density. In collaboration with Dr. Terry Udovic at NIST and Dr. Brandon Wood at LLNL, we have developed a suite of solid-state electrolytes based on polyboron clusters which display superionic conductivity, and elucidated the key structural, chemical, and dynamical factors that govern superionic ion transport in this class of materials.
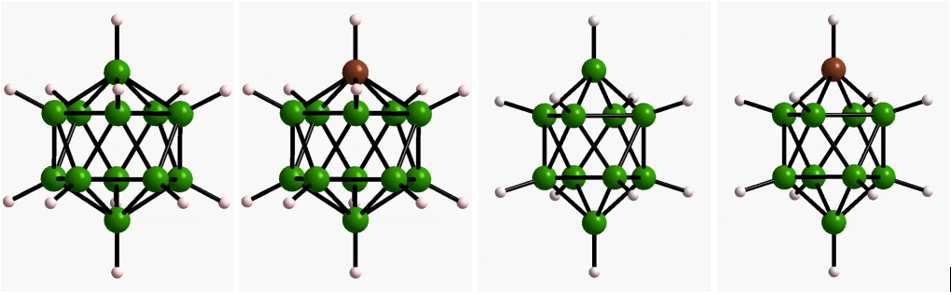
- Dimitrievska, M.; Shea, P.; Kweon, K. E.; Bercx, M.; Varley, J. B.; Tang, W. S.; Skripov, A. V.; Stavila, V.; Udovic, T. J.; Wood, B. C. Advanced Energy Materials 2018, 8, 1703422. ”Carbon Incorporation and Anion Dynamics as Synergistic Drivers for Ultrafast Diffusion in Superionic LiCB11H12 and NaCB11H12.”
- Tang, W. S.; Dimitrievska, M.; Stavila, V.; Zhou, W.; Wu, H.; Talin, A. A.; Udovic, T. J., Chemistry of Materials 2017, 29, 10496-10509. “Order-Disorder Transitions and Superionic Conductivity in the Sodium nido-Undeca(carba)borates.”
- Kweon, K. E.; Varley, J. B.; Shea, P.; Adelstein, N.; Mehta, P.; Heo, T. W.; Udovic, T. J.; Stavila, V.; Wood, B. C. Chemistry of Materials 2017, 29, 9142-9153. “Structural, Chemical, and Dynamical Frustration: Origins of Superionic Conductivity in closo-Borate Solid Electrolytes.”